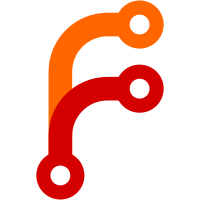
When an island contains exactly one infill ExtrusionEntity, this extrusion was oriented to maximize travel distance from the end of the previous extrusion of perimeters instead of minimizing it.
2047 lines
97 KiB
C++
2047 lines
97 KiB
C++
#if 0
|
|
#pragma optimize("", off)
|
|
#undef NDEBUG
|
|
#undef assert
|
|
#endif
|
|
|
|
#include "clipper.hpp"
|
|
#include "ShortestPath.hpp"
|
|
#include "KDTreeIndirect.hpp"
|
|
#include "MutablePriorityQueue.hpp"
|
|
#include "Print.hpp"
|
|
|
|
#include <cmath>
|
|
#include <cassert>
|
|
|
|
namespace Slic3r {
|
|
|
|
// Naive implementation of the Traveling Salesman Problem, it works by always taking the next closest neighbor.
|
|
// This implementation will always produce valid result even if some segments cannot reverse.
|
|
template<typename EndPointType, typename KDTreeType, typename CouldReverseFunc>
|
|
std::vector<std::pair<size_t, bool>> chain_segments_closest_point(std::vector<EndPointType> &end_points, KDTreeType &kdtree, CouldReverseFunc &could_reverse_func, EndPointType &first_point)
|
|
{
|
|
assert((end_points.size() & 1) == 0);
|
|
size_t num_segments = end_points.size() / 2;
|
|
assert(num_segments >= 2);
|
|
for (EndPointType &ep : end_points)
|
|
ep.chain_id = 0;
|
|
std::vector<std::pair<size_t, bool>> out;
|
|
out.reserve(num_segments);
|
|
size_t first_point_idx = &first_point - end_points.data();
|
|
out.emplace_back(first_point_idx / 2, (first_point_idx & 1) != 0);
|
|
first_point.chain_id = 1;
|
|
size_t this_idx = first_point_idx ^ 1;
|
|
for (int iter = (int)num_segments - 2; iter >= 0; -- iter) {
|
|
EndPointType &this_point = end_points[this_idx];
|
|
this_point.chain_id = 1;
|
|
// Find the closest point to this end_point, which lies on a different extrusion path (filtered by the lambda).
|
|
// Ignore the starting point as the starting point is considered to be occupied, no end point coud connect to it.
|
|
size_t next_idx = find_closest_point(kdtree, this_point.pos,
|
|
[this_idx, &end_points, &could_reverse_func](size_t idx) {
|
|
return (idx ^ this_idx) > 1 && end_points[idx].chain_id == 0 && ((idx & 1) == 0 || could_reverse_func(idx >> 1));
|
|
});
|
|
assert(next_idx < end_points.size());
|
|
EndPointType &end_point = end_points[next_idx];
|
|
end_point.chain_id = 1;
|
|
assert((next_idx & 1) == 0 || could_reverse_func(next_idx >> 1));
|
|
out.emplace_back(next_idx / 2, (next_idx & 1) != 0);
|
|
this_idx = next_idx ^ 1;
|
|
}
|
|
#ifndef NDEBUG
|
|
assert(end_points[this_idx].chain_id == 0);
|
|
for (EndPointType &ep : end_points)
|
|
assert(&ep == &end_points[this_idx] || ep.chain_id == 1);
|
|
#endif /* NDEBUG */
|
|
return out;
|
|
}
|
|
|
|
// Chain perimeters (always closed) and thin fills (closed or open) using a greedy algorithm.
|
|
// Solving a Traveling Salesman Problem (TSP) with the modification, that the sites are not always points, but points and segments.
|
|
// Solving using a greedy algorithm, where a shortest edge is added to the solution if it does not produce a bifurcation or a cycle.
|
|
// Return index and "reversed" flag.
|
|
// https://en.wikipedia.org/wiki/Multi-fragment_algorithm
|
|
// The algorithm builds a tour for the traveling salesman one edge at a time and thus maintains multiple tour fragments, each of which
|
|
// is a simple path in the complete graph of cities. At each stage, the algorithm selects the edge of minimal cost that either creates
|
|
// a new fragment, extends one of the existing paths or creates a cycle of length equal to the number of cities.
|
|
template<typename PointType, typename SegmentEndPointFunc, bool REVERSE_COULD_FAIL, typename CouldReverseFunc>
|
|
std::vector<std::pair<size_t, bool>> chain_segments_greedy_constrained_reversals_(SegmentEndPointFunc end_point_func, CouldReverseFunc could_reverse_func, size_t num_segments, const PointType *start_near)
|
|
{
|
|
std::vector<std::pair<size_t, bool>> out;
|
|
|
|
if (num_segments == 0) {
|
|
// Nothing to do.
|
|
}
|
|
else if (num_segments == 1)
|
|
{
|
|
// Just sort the end points so that the first point visited is closest to start_near.
|
|
out.emplace_back(0, could_reverse_func(0) && start_near != nullptr &&
|
|
(end_point_func(0, false) - *start_near).template cast<double>().squaredNorm() < (end_point_func(0, true) - *start_near).template cast<double>().squaredNorm());
|
|
}
|
|
else
|
|
{
|
|
// End points of segments for the KD tree closest point search.
|
|
// A single end point is inserted into the search structure for loops, two end points are entered for open paths.
|
|
struct EndPoint {
|
|
EndPoint(const Vec2d &pos) : pos(pos) {}
|
|
Vec2d pos;
|
|
// Identifier of the chain, to which this end point belongs. Zero means unassigned.
|
|
size_t chain_id = 0;
|
|
// Link to the closest currently valid end point.
|
|
EndPoint *edge_out = nullptr;
|
|
// Distance to the next end point following the link.
|
|
// Zero value -> start of the final path.
|
|
double distance_out = std::numeric_limits<double>::max();
|
|
size_t heap_idx = std::numeric_limits<size_t>::max();
|
|
};
|
|
std::vector<EndPoint> end_points;
|
|
end_points.reserve(num_segments * 2);
|
|
for (size_t i = 0; i < num_segments; ++ i) {
|
|
end_points.emplace_back(end_point_func(i, true ).template cast<double>());
|
|
end_points.emplace_back(end_point_func(i, false).template cast<double>());
|
|
}
|
|
|
|
// Construct the closest point KD tree over end points of segments.
|
|
auto coordinate_fn = [&end_points](size_t idx, size_t dimension) -> double { return end_points[idx].pos[dimension]; };
|
|
KDTreeIndirect<2, double, decltype(coordinate_fn)> kdtree(coordinate_fn, end_points.size());
|
|
|
|
// Helper to detect loops in already connected paths.
|
|
// Unique chain IDs are assigned to paths. If paths are connected, end points will not have their chain IDs updated, but the chain IDs
|
|
// will remember an "equivalent" chain ID, which is the lowest ID of all the IDs in the path, and the lowest ID is equivalent to itself.
|
|
class EquivalentChains {
|
|
public:
|
|
// Zero'th chain ID is invalid.
|
|
EquivalentChains(size_t reserve) { m_equivalent_with.reserve(reserve); m_equivalent_with.emplace_back(0); }
|
|
// Generate next equivalence class.
|
|
size_t next() {
|
|
m_equivalent_with.emplace_back(++ m_last_chain_id);
|
|
return m_last_chain_id;
|
|
}
|
|
// Get equivalence class for chain ID.
|
|
size_t operator()(size_t chain_id) {
|
|
if (chain_id != 0) {
|
|
for (size_t last = chain_id;;) {
|
|
size_t lower = m_equivalent_with[last];
|
|
if (lower == last) {
|
|
m_equivalent_with[chain_id] = lower;
|
|
chain_id = lower;
|
|
break;
|
|
}
|
|
last = lower;
|
|
}
|
|
}
|
|
return chain_id;
|
|
}
|
|
size_t merge(size_t chain_id1, size_t chain_id2) {
|
|
size_t chain_id = std::min((*this)(chain_id1), (*this)(chain_id2));
|
|
m_equivalent_with[chain_id1] = chain_id;
|
|
m_equivalent_with[chain_id2] = chain_id;
|
|
return chain_id;
|
|
}
|
|
|
|
#ifndef NDEBUG
|
|
bool validate()
|
|
{
|
|
assert(m_last_chain_id >= 0);
|
|
assert(m_last_chain_id + 1 == m_equivalent_with.size());
|
|
for (size_t i = 0; i < m_equivalent_with.size(); ++ i) {
|
|
for (size_t last = i;;) {
|
|
size_t lower = m_equivalent_with[last];
|
|
assert(lower <= last);
|
|
if (lower == last)
|
|
break;
|
|
last = lower;
|
|
}
|
|
}
|
|
return true;
|
|
}
|
|
#endif /* NDEBUG */
|
|
|
|
private:
|
|
// Unique chain ID assigned to chains of end points of segments.
|
|
size_t m_last_chain_id = 0;
|
|
std::vector<size_t> m_equivalent_with;
|
|
} equivalent_chain(num_segments);
|
|
|
|
// Find the first end point closest to start_near.
|
|
EndPoint *first_point = nullptr;
|
|
size_t first_point_idx = std::numeric_limits<size_t>::max();
|
|
if (start_near != nullptr) {
|
|
size_t idx = find_closest_point(kdtree, start_near->template cast<double>(),
|
|
// Don't start with a reverse segment, if flipping of the segment is not allowed.
|
|
[&could_reverse_func](size_t idx) { return (idx & 1) == 0 || could_reverse_func(idx >> 1); });
|
|
assert(idx < end_points.size());
|
|
first_point = &end_points[idx];
|
|
first_point->distance_out = 0.;
|
|
first_point->chain_id = equivalent_chain.next();
|
|
first_point_idx = idx;
|
|
}
|
|
EndPoint *initial_point = first_point;
|
|
EndPoint *last_point = nullptr;
|
|
|
|
// Assign the closest point and distance to the end points.
|
|
for (EndPoint &end_point : end_points) {
|
|
assert(end_point.edge_out == nullptr);
|
|
if (&end_point != first_point) {
|
|
size_t this_idx = &end_point - &end_points.front();
|
|
// Find the closest point to this end_point, which lies on a different extrusion path (filtered by the lambda).
|
|
// Ignore the starting point as the starting point is considered to be occupied, no end point coud connect to it.
|
|
size_t next_idx = find_closest_point(kdtree, end_point.pos,
|
|
[this_idx, first_point_idx](size_t idx){ return idx != first_point_idx && (idx ^ this_idx) > 1; });
|
|
assert(next_idx < end_points.size());
|
|
EndPoint &end_point2 = end_points[next_idx];
|
|
end_point.edge_out = &end_point2;
|
|
end_point.distance_out = (end_point2.pos - end_point.pos).squaredNorm();
|
|
}
|
|
}
|
|
|
|
// Initialize a heap of end points sorted by the lowest distance to the next valid point of a path.
|
|
auto queue = make_mutable_priority_queue<EndPoint*, false>(
|
|
[](EndPoint *ep, size_t idx){ ep->heap_idx = idx; },
|
|
[](EndPoint *l, EndPoint *r){ return l->distance_out < r->distance_out; });
|
|
queue.reserve(end_points.size() * 2 - 1);
|
|
for (EndPoint &ep : end_points)
|
|
if (first_point != &ep)
|
|
queue.push(&ep);
|
|
|
|
#ifndef NDEBUG
|
|
auto validate_graph_and_queue = [&equivalent_chain, &end_points, &queue, first_point]() -> bool {
|
|
assert(equivalent_chain.validate());
|
|
for (EndPoint &ep : end_points) {
|
|
if (ep.heap_idx < queue.size()) {
|
|
// End point is on the heap.
|
|
assert(*(queue.cbegin() + ep.heap_idx) == &ep);
|
|
assert(ep.chain_id == 0);
|
|
} else {
|
|
// End point is NOT on the heap, therefore it is part of the output path.
|
|
assert(ep.heap_idx == std::numeric_limits<size_t>::max());
|
|
assert(ep.chain_id != 0);
|
|
if (&ep == first_point) {
|
|
assert(ep.edge_out == nullptr);
|
|
} else {
|
|
assert(ep.edge_out != nullptr);
|
|
// Detect loops.
|
|
for (EndPoint *pt = &ep; pt != nullptr;) {
|
|
// Out of queue. It is a final point.
|
|
assert(pt->heap_idx == std::numeric_limits<size_t>::max());
|
|
EndPoint *pt_other = &end_points[(pt - &end_points.front()) ^ 1];
|
|
if (pt_other->heap_idx < queue.size())
|
|
// The other side of this segment is undecided yet.
|
|
break;
|
|
pt = pt_other->edge_out;
|
|
}
|
|
}
|
|
}
|
|
}
|
|
for (EndPoint *ep : queue)
|
|
// Points in the queue are not connected yet.
|
|
assert(ep->chain_id == 0);
|
|
return true;
|
|
};
|
|
#endif /* NDEBUG */
|
|
|
|
// Chain the end points: find (num_segments - 1) shortest links not forming bifurcations or loops.
|
|
assert(num_segments >= 2);
|
|
#ifndef NDEBUG
|
|
double distance_taken_last = 0.;
|
|
#endif /* NDEBUG */
|
|
for (int iter = int(num_segments) - 2;; -- iter) {
|
|
assert(validate_graph_and_queue());
|
|
// Take the first end point, for which the link points to the currently closest valid neighbor.
|
|
EndPoint &end_point1 = *queue.top();
|
|
#ifndef NDEBUG
|
|
// Each edge added shall be longer than the previous one taken.
|
|
assert(end_point1.distance_out > distance_taken_last - SCALED_EPSILON);
|
|
distance_taken_last = end_point1.distance_out;
|
|
#endif /* NDEBUG */
|
|
assert(end_point1.edge_out != nullptr);
|
|
// No point on the queue may be connected yet.
|
|
assert(end_point1.chain_id == 0);
|
|
// Take the closest end point to the first end point,
|
|
EndPoint &end_point2 = *end_point1.edge_out;
|
|
bool valid = true;
|
|
size_t end_point1_other_chain_id = 0;
|
|
size_t end_point2_other_chain_id = 0;
|
|
if (end_point2.chain_id > 0) {
|
|
// The other side is part of the output path. Don't connect to end_point2, update end_point1 and try another one.
|
|
valid = false;
|
|
} else {
|
|
// End points of the opposite ends of the segments.
|
|
end_point1_other_chain_id = equivalent_chain(end_points[(&end_point1 - &end_points.front()) ^ 1].chain_id);
|
|
end_point2_other_chain_id = equivalent_chain(end_points[(&end_point2 - &end_points.front()) ^ 1].chain_id);
|
|
if (end_point1_other_chain_id == end_point2_other_chain_id && end_point1_other_chain_id != 0)
|
|
// This edge forms a loop. Update end_point1 and try another one.
|
|
valid = false;
|
|
}
|
|
if (valid) {
|
|
// Remove the first and second point from the queue.
|
|
queue.pop();
|
|
queue.remove(end_point2.heap_idx);
|
|
assert(end_point1.edge_out = &end_point2);
|
|
end_point2.edge_out = &end_point1;
|
|
end_point2.distance_out = end_point1.distance_out;
|
|
// Assign chain IDs to the newly connected end points, set equivalent_chain if two chains were merged.
|
|
size_t chain_id =
|
|
(end_point1_other_chain_id == 0) ?
|
|
((end_point2_other_chain_id == 0) ? equivalent_chain.next() : end_point2_other_chain_id) :
|
|
((end_point2_other_chain_id == 0) ? end_point1_other_chain_id :
|
|
(end_point1_other_chain_id == end_point2_other_chain_id) ?
|
|
end_point1_other_chain_id :
|
|
equivalent_chain.merge(end_point1_other_chain_id, end_point2_other_chain_id));
|
|
end_point1.chain_id = chain_id;
|
|
end_point2.chain_id = chain_id;
|
|
assert(validate_graph_and_queue());
|
|
if (iter == 0) {
|
|
// Last iteration. There shall be exactly one or two end points waiting to be connected.
|
|
assert(queue.size() == ((first_point == nullptr) ? 2 : 1));
|
|
if (first_point == nullptr) {
|
|
first_point = queue.top();
|
|
queue.pop();
|
|
first_point->edge_out = nullptr;
|
|
}
|
|
last_point = queue.top();
|
|
last_point->edge_out = nullptr;
|
|
queue.pop();
|
|
assert(queue.empty());
|
|
break;
|
|
}
|
|
} else {
|
|
// This edge forms a loop. Update end_point1 and try another one.
|
|
++ iter;
|
|
end_point1.edge_out = nullptr;
|
|
// Update edge_out and distance.
|
|
size_t this_idx = &end_point1 - &end_points.front();
|
|
// Find the closest point to this end_point, which lies on a different extrusion path (filtered by the filter lambda).
|
|
size_t next_idx = find_closest_point(kdtree, end_point1.pos, [&end_points, &equivalent_chain, this_idx](size_t idx) {
|
|
assert(end_points[this_idx].edge_out == nullptr);
|
|
assert(end_points[this_idx].chain_id == 0);
|
|
if ((idx ^ this_idx) <= 1 || end_points[idx].chain_id != 0)
|
|
// Points of the same segment shall not be connected,
|
|
// cannot connect to an already connected point (ideally those would be removed from the KD tree, but the update is difficult).
|
|
return false;
|
|
size_t chain1 = equivalent_chain(end_points[this_idx ^ 1].chain_id);
|
|
size_t chain2 = equivalent_chain(end_points[idx ^ 1].chain_id);
|
|
return chain1 != chain2 || chain1 == 0;
|
|
});
|
|
assert(next_idx < end_points.size());
|
|
end_point1.edge_out = &end_points[next_idx];
|
|
end_point1.distance_out = (end_points[next_idx].pos - end_point1.pos).squaredNorm();
|
|
#ifndef NDEBUG
|
|
// Each edge shall be longer than the last one removed from the queue.
|
|
assert(end_point1.distance_out > distance_taken_last - SCALED_EPSILON);
|
|
#endif /* NDEBUG */
|
|
// Update position of this end point in the queue based on the distance calculated at the line above.
|
|
queue.update(end_point1.heap_idx);
|
|
//FIXME Remove the other end point from the KD tree.
|
|
// As the KD tree update is expensive, do it only after some larger number of points is removed from the queue.
|
|
assert(validate_graph_and_queue());
|
|
}
|
|
}
|
|
assert(queue.empty());
|
|
|
|
// Now interconnect pairs of segments into a chain.
|
|
assert(first_point != nullptr);
|
|
out.reserve(num_segments);
|
|
bool failed = false;
|
|
do {
|
|
assert(out.size() < num_segments);
|
|
size_t first_point_id = first_point - &end_points.front();
|
|
size_t segment_id = first_point_id >> 1;
|
|
bool reverse = (first_point_id & 1) != 0;
|
|
EndPoint *second_point = &end_points[first_point_id ^ 1];
|
|
if (REVERSE_COULD_FAIL) {
|
|
if (reverse && ! could_reverse_func(segment_id)) {
|
|
failed = true;
|
|
break;
|
|
}
|
|
} else {
|
|
assert(! reverse || could_reverse_func(segment_id));
|
|
}
|
|
out.emplace_back(segment_id, reverse);
|
|
first_point = second_point->edge_out;
|
|
} while (first_point != nullptr);
|
|
if (REVERSE_COULD_FAIL) {
|
|
if (failed) {
|
|
if (start_near == nullptr) {
|
|
// We may try the reverse order.
|
|
out.clear();
|
|
first_point = last_point;
|
|
failed = false;
|
|
do {
|
|
assert(out.size() < num_segments);
|
|
size_t first_point_id = first_point - &end_points.front();
|
|
size_t segment_id = first_point_id >> 1;
|
|
bool reverse = (first_point_id & 1) != 0;
|
|
EndPoint *second_point = &end_points[first_point_id ^ 1];
|
|
if (reverse && ! could_reverse_func(segment_id)) {
|
|
failed = true;
|
|
break;
|
|
}
|
|
out.emplace_back(segment_id, reverse);
|
|
first_point = second_point->edge_out;
|
|
} while (first_point != nullptr);
|
|
}
|
|
}
|
|
if (failed)
|
|
// As a last resort, try a dumb algorithm, which is not sensitive to edge reversal constraints.
|
|
out = chain_segments_closest_point<EndPoint, decltype(kdtree), CouldReverseFunc>(end_points, kdtree, could_reverse_func, (initial_point != nullptr) ? *initial_point : end_points.front());
|
|
} else {
|
|
assert(! failed);
|
|
}
|
|
}
|
|
|
|
assert(out.size() == num_segments);
|
|
return out;
|
|
}
|
|
|
|
template<typename QueueType, typename KDTreeType, typename ChainsType, typename EndPointType>
|
|
void update_end_point_in_queue(QueueType &queue, const KDTreeType &kdtree, ChainsType &chains, std::vector<EndPointType> &end_points, EndPointType &end_point, size_t first_point_idx, const EndPointType *first_point)
|
|
{
|
|
// Updating an end point or a 2nd from an end point.
|
|
size_t this_idx = end_point.index(end_points);
|
|
// If this segment is not the starting segment, then this end point or the opposite is unconnected.
|
|
assert(first_point_idx == this_idx || first_point_idx == (this_idx ^ 1) || end_point.chain_id == 0 || end_point.opposite(end_points).chain_id == 0);
|
|
end_point.edge_candidate = nullptr;
|
|
if (first_point_idx == this_idx || (end_point.chain_id > 0 && first_point_idx == (this_idx ^ 1)))
|
|
{
|
|
// One may never flip the 1st edge, don't try it again.
|
|
if (! end_point.heap_idx_invalid())
|
|
queue.remove(end_point.heap_idx);
|
|
}
|
|
else
|
|
{
|
|
// Update edge_candidate and distance.
|
|
size_t chain1a = end_point.chain_id;
|
|
size_t chain1b = end_points[this_idx ^ 1].chain_id;
|
|
size_t this_chain = chains.equivalent(std::max(chain1a, chain1b));
|
|
// Find the closest point to this end_point, which lies on a different extrusion path (filtered by the filter lambda).
|
|
size_t next_idx = find_closest_point(kdtree, end_point.pos, [&end_points, &chains, this_idx, first_point_idx, first_point, this_chain](size_t idx) {
|
|
assert(end_points[this_idx].edge_candidate == nullptr);
|
|
// Either this end of the edge or the other end of the edge is not yet connected.
|
|
assert((end_points[this_idx ].chain_id == 0 && end_points[this_idx ].edge_out == nullptr) ||
|
|
(end_points[this_idx ^ 1].chain_id == 0 && end_points[this_idx ^ 1].edge_out == nullptr));
|
|
if ((idx ^ this_idx) <= 1 || idx == first_point_idx)
|
|
// Points of the same segment shall not be connected.
|
|
// Don't connect to the first point, we must not flip the 1st edge.
|
|
return false;
|
|
size_t chain2a = end_points[idx].chain_id;
|
|
size_t chain2b = end_points[idx ^ 1].chain_id;
|
|
if (chain2a > 0 && chain2b > 0)
|
|
// Only unconnected end point or a point next to an unconnected end point may be connected to.
|
|
// Ideally those would be removed from the KD tree, but the update is difficult.
|
|
return false;
|
|
assert(chain2a == 0 || chain2b == 0);
|
|
size_t chain2 = chains.equivalent(std::max(chain2a, chain2b));
|
|
if (this_chain == chain2)
|
|
// Don't connect back to the same chain, don't create a loop.
|
|
return this_chain == 0;
|
|
// Don't connect to a segment requiring flipping if the segment starts or ends with the first point.
|
|
if (chain2a > 0) {
|
|
// Chain requires flipping.
|
|
assert(chain2b == 0);
|
|
auto &chain = chains.chain(chain2);
|
|
if (chain.begin == first_point || chain.end == first_point)
|
|
return false;
|
|
}
|
|
// Everything is all right, try to connect.
|
|
return true;
|
|
});
|
|
assert(next_idx < end_points.size());
|
|
assert(chains.equivalent(end_points[next_idx].chain_id) != chains.equivalent(end_points[next_idx ^ 1].chain_id) || end_points[next_idx].chain_id == 0);
|
|
end_point.edge_candidate = &end_points[next_idx];
|
|
end_point.distance_out = (end_points[next_idx].pos - end_point.pos).norm();
|
|
if (end_point.chain_id > 0)
|
|
end_point.distance_out += chains.chain_flip_penalty(this_chain);
|
|
if (end_points[next_idx].chain_id > 0)
|
|
// The candidate chain is flipped.
|
|
end_point.distance_out += chains.chain_flip_penalty(end_points[next_idx].chain_id);
|
|
// Update position of this end point in the queue based on the distance calculated at the line above.
|
|
if (end_point.heap_idx_invalid())
|
|
queue.push(&end_point);
|
|
else
|
|
queue.update(end_point.heap_idx);
|
|
}
|
|
}
|
|
|
|
template<typename PointType, typename SegmentEndPointFunc, bool REVERSE_COULD_FAIL, typename CouldReverseFunc>
|
|
std::vector<std::pair<size_t, bool>> chain_segments_greedy_constrained_reversals2_(SegmentEndPointFunc end_point_func, CouldReverseFunc could_reverse_func, size_t num_segments, const PointType *start_near)
|
|
{
|
|
std::vector<std::pair<size_t, bool>> out;
|
|
|
|
if (num_segments == 0) {
|
|
// Nothing to do.
|
|
}
|
|
else if (num_segments == 1)
|
|
{
|
|
// Just sort the end points so that the first point visited is closest to start_near.
|
|
out.emplace_back(0, start_near != nullptr &&
|
|
(end_point_func(0, true) - *start_near).template cast<double>().squaredNorm() < (end_point_func(0, false) - *start_near).template cast<double>().squaredNorm());
|
|
}
|
|
else
|
|
{
|
|
// End points of segments for the KD tree closest point search.
|
|
// A single end point is inserted into the search structure for loops, two end points are entered for open paths.
|
|
struct EndPoint {
|
|
EndPoint(const Vec2d &pos) : pos(pos) {}
|
|
Vec2d pos;
|
|
|
|
// Candidate for a new connection link.
|
|
EndPoint *edge_candidate = nullptr;
|
|
// Distance to the next end point following the link.
|
|
// Zero value -> start of the final path.
|
|
double distance_out = std::numeric_limits<double>::max();
|
|
|
|
size_t heap_idx = std::numeric_limits<size_t>::max();
|
|
bool heap_idx_invalid() const { return this->heap_idx == std::numeric_limits<size_t>::max(); }
|
|
|
|
// Identifier of the chain, to which this end point belongs. Zero means unassigned.
|
|
size_t chain_id = 0;
|
|
// Double linked chain of segment end points in current path.
|
|
EndPoint *edge_out = nullptr;
|
|
|
|
size_t index(std::vector<EndPoint> &endpoints) const { return this - endpoints.data(); }
|
|
// Opposite end point of the same segment.
|
|
EndPoint& opposite(std::vector<EndPoint> &endpoints) { return endpoints[(this - endpoints.data()) ^ 1]; }
|
|
const EndPoint& opposite(const std::vector<EndPoint> &endpoints) const { return endpoints[(this - endpoints.data()) ^ 1]; }
|
|
};
|
|
|
|
std::vector<EndPoint> end_points;
|
|
end_points.reserve(num_segments * 2);
|
|
for (size_t i = 0; i < num_segments; ++ i) {
|
|
end_points.emplace_back(end_point_func(i, true ).template cast<double>());
|
|
end_points.emplace_back(end_point_func(i, false).template cast<double>());
|
|
}
|
|
|
|
// Construct the closest point KD tree over end points of segments.
|
|
auto coordinate_fn = [&end_points](size_t idx, size_t dimension) -> double { return end_points[idx].pos[dimension]; };
|
|
KDTreeIndirect<2, double, decltype(coordinate_fn)> kdtree(coordinate_fn, end_points.size());
|
|
|
|
// Chained segments with their sum of connection lengths.
|
|
// The chain supports flipping all the segments, connecting the segments at the opposite ends.
|
|
// (this is a very useful path optimization for infill lines).
|
|
struct Chain {
|
|
size_t num_segments = 0;
|
|
double cost = 0.;
|
|
double cost_flipped = 0.;
|
|
EndPoint *begin = nullptr;
|
|
EndPoint *end = nullptr;
|
|
size_t equivalent_with = 0;
|
|
|
|
// Flipping the chain has a time complexity of O(n).
|
|
void flip(std::vector<EndPoint> &endpoints)
|
|
{
|
|
assert(this->num_segments > 1);
|
|
assert(this->begin->edge_out == nullptr);
|
|
assert(this->end ->edge_out == nullptr);
|
|
assert(this->begin->opposite(endpoints).edge_out != nullptr);
|
|
assert(this->end ->opposite(endpoints).edge_out != nullptr);
|
|
// Start of the current segment processed.
|
|
EndPoint *ept = this->begin;
|
|
// Previous end point to connect the other side of ept to.
|
|
EndPoint *ept_prev = nullptr;
|
|
do {
|
|
EndPoint *ept_end = &ept->opposite(endpoints);
|
|
EndPoint *ept_next = ept_end->edge_out;
|
|
assert(ept_next == nullptr || ept_next->edge_out == ept_end);
|
|
// Connect to the preceding segment.
|
|
ept_end->edge_out = ept_prev;
|
|
if (ept_prev != nullptr)
|
|
ept_prev->edge_out = ept_end;
|
|
ept_prev = ept;
|
|
ept = ept_next;
|
|
} while (ept != nullptr);
|
|
ept_prev->edge_out = nullptr;
|
|
// Swap the costs.
|
|
std::swap(this->cost, this->cost_flipped);
|
|
// Swap the ends.
|
|
EndPoint *new_begin = &this->begin->opposite(endpoints);
|
|
EndPoint *new_end = &this->end->opposite(endpoints);
|
|
std::swap(this->begin->chain_id, new_begin->chain_id);
|
|
std::swap(this->end ->chain_id, new_end ->chain_id);
|
|
this->begin = new_begin;
|
|
this->end = new_end;
|
|
assert(this->begin->edge_out == nullptr);
|
|
assert(this->end ->edge_out == nullptr);
|
|
assert(this->begin->opposite(endpoints).edge_out != nullptr);
|
|
assert(this->end ->opposite(endpoints).edge_out != nullptr);
|
|
}
|
|
|
|
double flip_penalty() const { return this->cost_flipped - this->cost; }
|
|
};
|
|
|
|
// Helper to detect loops in already connected paths and to accomodate flipping of chains.
|
|
//
|
|
// Unique chain IDs are assigned to paths. If paths are connected, end points will not have their chain IDs updated, but the chain IDs
|
|
// will remember an "equivalent" chain ID, which is the lowest ID of all the IDs in the path, and the lowest ID is equivalent to itself.
|
|
// Chain IDs are indexed starting with 1.
|
|
//
|
|
// Chains remember their lengths and their lengths when each segment of the chain is flipped.
|
|
class Chains {
|
|
public:
|
|
// Zero'th chain ID is invalid.
|
|
Chains(size_t reserve) {
|
|
m_chains.reserve(reserve / 2);
|
|
// Indexing starts with 1.
|
|
m_chains.emplace_back();
|
|
}
|
|
|
|
// Generate next equivalence class.
|
|
size_t next_id() {
|
|
m_chains.emplace_back();
|
|
m_chains.back().equivalent_with = ++ m_last_chain_id;
|
|
return m_last_chain_id;
|
|
}
|
|
|
|
// Get equivalence class for chain ID, update the "equivalent_with" along the equivalence path.
|
|
size_t equivalent(size_t chain_id) {
|
|
if (chain_id != 0) {
|
|
for (size_t last = chain_id;;) {
|
|
size_t lower = m_chains[last].equivalent_with;
|
|
if (lower == last) {
|
|
m_chains[chain_id].equivalent_with = lower;
|
|
chain_id = lower;
|
|
break;
|
|
}
|
|
last = lower;
|
|
}
|
|
}
|
|
return chain_id;
|
|
}
|
|
|
|
// Return a lowest chain ID of the two input chains.
|
|
// Produce a new chain ID of both chain IDs are zero.
|
|
size_t merge(size_t chain_id1, size_t chain_id2) {
|
|
if (chain_id1 == 0)
|
|
return (chain_id2 == 0) ? this->next_id() : chain_id2;
|
|
if (chain_id2 == 0)
|
|
return chain_id1;
|
|
assert(m_chains[chain_id1].equivalent_with == chain_id1);
|
|
assert(m_chains[chain_id2].equivalent_with == chain_id2);
|
|
size_t chain_id = std::min(chain_id1, chain_id2);
|
|
m_chains[chain_id1].equivalent_with = chain_id;
|
|
m_chains[chain_id2].equivalent_with = chain_id;
|
|
return chain_id;
|
|
}
|
|
|
|
Chain& chain(size_t chain_id) { return m_chains[chain_id]; }
|
|
const Chain& chain(size_t chain_id) const { return m_chains[chain_id]; }
|
|
|
|
double chain_flip_penalty(size_t chain_id) {
|
|
chain_id = this->equivalent(chain_id);
|
|
return m_chains[chain_id].flip_penalty();
|
|
}
|
|
|
|
#ifndef NDEBUG
|
|
bool validate()
|
|
{
|
|
// Validate that the segments merged chain IDs make up a directed acyclic graph
|
|
// with edges oriented towards the lower chain ID, therefore all ending up
|
|
// in the lowest chain ID of all of them.
|
|
assert(m_last_chain_id >= 0);
|
|
assert(m_last_chain_id + 1 == m_chains.size());
|
|
for (size_t i = 0; i < m_chains.size(); ++ i) {
|
|
for (size_t last = i;;) {
|
|
size_t lower = m_chains[last].equivalent_with;
|
|
assert(lower <= last);
|
|
if (lower == last)
|
|
break;
|
|
last = lower;
|
|
}
|
|
}
|
|
return true;
|
|
}
|
|
#endif /* NDEBUG */
|
|
|
|
private:
|
|
std::vector<Chain> m_chains;
|
|
// Unique chain ID assigned to chains of end points of segments.
|
|
size_t m_last_chain_id = 0;
|
|
} chains(num_segments);
|
|
|
|
// Find the first end point closest to start_near.
|
|
EndPoint *first_point = nullptr;
|
|
size_t first_point_idx = std::numeric_limits<size_t>::max();
|
|
if (start_near != nullptr) {
|
|
size_t idx = find_closest_point(kdtree, start_near->template cast<double>());
|
|
assert(idx < end_points.size());
|
|
first_point = &end_points[idx];
|
|
first_point->distance_out = 0.;
|
|
first_point->chain_id = chains.next_id();
|
|
Chain &chain = chains.chain(first_point->chain_id);
|
|
chain.begin = first_point;
|
|
chain.end = &first_point->opposite(end_points);
|
|
first_point_idx = idx;
|
|
}
|
|
EndPoint *initial_point = first_point;
|
|
EndPoint *last_point = nullptr;
|
|
|
|
// Assign the closest point and distance to the end points.
|
|
for (EndPoint &end_point : end_points) {
|
|
assert(end_point.edge_candidate == nullptr);
|
|
if (&end_point != first_point) {
|
|
size_t this_idx = end_point.index(end_points);
|
|
// Find the closest point to this end_point, which lies on a different extrusion path (filtered by the lambda).
|
|
// Ignore the starting point as the starting point is considered to be occupied, no end point coud connect to it.
|
|
size_t next_idx = find_closest_point(kdtree, end_point.pos,
|
|
[this_idx, first_point_idx](size_t idx){ return idx != first_point_idx && (idx ^ this_idx) > 1; });
|
|
assert(next_idx < end_points.size());
|
|
EndPoint &end_point2 = end_points[next_idx];
|
|
end_point.edge_candidate = &end_point2;
|
|
end_point.distance_out = (end_point2.pos - end_point.pos).norm();
|
|
}
|
|
}
|
|
|
|
// Initialize a heap of end points sorted by the lowest distance to the next valid point of a path.
|
|
auto queue = make_mutable_priority_queue<EndPoint*, true>(
|
|
[](EndPoint *ep, size_t idx){ ep->heap_idx = idx; },
|
|
[](EndPoint *l, EndPoint *r){ return l->distance_out < r->distance_out; });
|
|
queue.reserve(end_points.size() * 2);
|
|
for (EndPoint &ep : end_points)
|
|
if (first_point != &ep)
|
|
queue.push(&ep);
|
|
|
|
#ifndef NDEBUG
|
|
auto validate_graph_and_queue = [&chains, &end_points, &queue, first_point]() -> bool {
|
|
assert(chains.validate());
|
|
for (EndPoint &ep : end_points) {
|
|
if (ep.heap_idx < queue.size()) {
|
|
// End point is on the heap.
|
|
assert(*(queue.cbegin() + ep.heap_idx) == &ep);
|
|
// One side or the other of the segment is not yet connected.
|
|
assert(ep.chain_id == 0 || ep.opposite(end_points).chain_id == 0);
|
|
} else {
|
|
// End point is NOT on the heap, therefore it must part of the output path.
|
|
assert(ep.heap_idx_invalid());
|
|
assert(ep.chain_id != 0);
|
|
if (&ep == first_point) {
|
|
assert(ep.edge_out == nullptr);
|
|
} else {
|
|
assert(ep.edge_out != nullptr);
|
|
// Detect loops.
|
|
for (EndPoint *pt = &ep; pt != nullptr;) {
|
|
// Out of queue. It is a final point.
|
|
EndPoint *pt_other = &pt->opposite(end_points);
|
|
if (pt_other->heap_idx < queue.size()) {
|
|
// The other side of this segment is undecided yet.
|
|
// assert(pt_other->edge_out == nullptr);
|
|
break;
|
|
}
|
|
pt = pt_other->edge_out;
|
|
}
|
|
}
|
|
}
|
|
}
|
|
for (EndPoint *ep : queue)
|
|
// Points in the queue or the opposites of the same segment are not connected yet.
|
|
assert(ep->chain_id == 0 || ep->opposite(end_points).chain_id == 0);
|
|
return true;
|
|
};
|
|
#endif /* NDEBUG */
|
|
|
|
// Chain the end points: find (num_segments - 1) shortest links not forming bifurcations or loops.
|
|
assert(num_segments >= 2);
|
|
#ifndef NDEBUG
|
|
double distance_taken_last = 0.;
|
|
#endif /* NDEBUG */
|
|
// Some links stored onto the priority queue are being invalidated during the calculation and they are not
|
|
// updated immediately. If such a situation is detected for an end point pulled from the priority queue,
|
|
// the end point is being updated and re-inserted into the priority queue. Therefore the number of iterations
|
|
// required is higher than expected (it would be the number of links, num_segments - 1).
|
|
// The limit here may not be necessary, but it guards us against an endless loop if something goes wrong.
|
|
size_t num_iter = num_segments * 16;
|
|
for (size_t num_connections_to_end = num_segments - 1; num_iter > 0; -- num_iter) {
|
|
assert(validate_graph_and_queue());
|
|
// Take the first end point, for which the link points to the currently closest valid neighbor.
|
|
EndPoint *end_point1 = queue.top();
|
|
assert(end_point1 != first_point);
|
|
EndPoint *end_point1_other = &end_point1->opposite(end_points);
|
|
// true if end_point1 is not the end of its chain, but the 2nd point. When connecting to the 2nd point, this chain needs
|
|
// to be flipped first.
|
|
bool chain1_flip = end_point1->chain_id > 0;
|
|
// Either this point at the queue is not connected, or it is the 2nd point of a chain.
|
|
// If connecting to a 2nd point of a chain, the 1st point shall not yet be connected and this chain will need
|
|
// to be flipped.
|
|
assert( chain1_flip || (end_point1->chain_id == 0 && end_point1->edge_out == nullptr));
|
|
assert(! chain1_flip || (end_point1_other->chain_id == 0 && end_point1_other->edge_out == nullptr));
|
|
assert(end_point1->edge_candidate != nullptr);
|
|
#ifndef NDEBUG
|
|
// Each edge added shall be longer than the previous one taken.
|
|
//assert(end_point1->distance_out > distance_taken_last - SCALED_EPSILON);
|
|
if (end_point1->distance_out < distance_taken_last - SCALED_EPSILON) {
|
|
// printf("Warning: taking shorter length than previously is suspicious\n");
|
|
}
|
|
distance_taken_last = end_point1->distance_out;
|
|
#endif /* NDEBUG */
|
|
// Take the closest end point to the first end point,
|
|
EndPoint *end_point2 = end_point1->edge_candidate;
|
|
EndPoint *end_point2_other = &end_point2->opposite(end_points);
|
|
bool chain2_flip = end_point2->chain_id > 0;
|
|
// Is the link from end_point1 to end_point2 still valid? If yes, the link may be taken. Otherwise the link needs to be refreshed.
|
|
bool valid = true;
|
|
size_t end_point1_chain_id = 0;
|
|
size_t end_point2_chain_id = 0;
|
|
if (end_point2->chain_id > 0 && end_point2_other->chain_id > 0) {
|
|
// The other side is part of the output path. Don't connect to end_point2, update end_point1 and try another one.
|
|
valid = false;
|
|
} else {
|
|
// End points of the opposite ends of the segments.
|
|
end_point1_chain_id = chains.equivalent((chain1_flip ? end_point1 : end_point1_other)->chain_id);
|
|
end_point2_chain_id = chains.equivalent((chain2_flip ? end_point2 : end_point2_other)->chain_id);
|
|
if (end_point1_chain_id == end_point2_chain_id && end_point1_chain_id != 0)
|
|
// This edge forms a loop. Update end_point1 and try another one.
|
|
valid = false;
|
|
else {
|
|
// Verify whether end_point1.distance_out still matches the current state of the two end points to be connected and their chains.
|
|
// Namely, the other chain may have been flipped in the meantime.
|
|
double dist = (end_point2->pos - end_point1->pos).norm();
|
|
if (chain1_flip)
|
|
dist += chains.chain_flip_penalty(end_point1_chain_id);
|
|
if (chain2_flip)
|
|
dist += chains.chain_flip_penalty(end_point2_chain_id);
|
|
if (std::abs(dist - end_point1->distance_out) > SCALED_EPSILON)
|
|
// The distance changed due to flipping of one of the chains. Refresh this end point in the queue.
|
|
valid = false;
|
|
}
|
|
if (valid && first_point != nullptr) {
|
|
// Verify that a chain starting or ending with the first_point does not get flipped.
|
|
if (chain1_flip) {
|
|
Chain &chain = chains.chain(end_point1_chain_id);
|
|
if (chain.begin == first_point || chain.end == first_point)
|
|
valid = false;
|
|
}
|
|
if (valid && chain2_flip) {
|
|
Chain &chain = chains.chain(end_point2_chain_id);
|
|
if (chain.begin == first_point || chain.end == first_point)
|
|
valid = false;
|
|
}
|
|
}
|
|
}
|
|
if (valid) {
|
|
// Remove the first and second point from the queue.
|
|
queue.pop();
|
|
queue.remove(end_point2->heap_idx);
|
|
assert(end_point1->edge_candidate == end_point2);
|
|
end_point1->edge_candidate = nullptr;
|
|
Chain *chain1 = (end_point1_chain_id == 0) ? nullptr : &chains.chain(end_point1_chain_id);
|
|
Chain *chain2 = (end_point2_chain_id == 0) ? nullptr : &chains.chain(end_point2_chain_id);
|
|
assert(chain1 == nullptr || (chain1_flip ? (chain1->begin == end_point1_other || chain1->end == end_point1_other) : (chain1->begin == end_point1 || chain1->end == end_point1)));
|
|
assert(chain2 == nullptr || (chain2_flip ? (chain2->begin == end_point2_other || chain2->end == end_point2_other) : (chain2->begin == end_point2 || chain2->end == end_point2)));
|
|
if (chain1_flip)
|
|
chain1->flip(end_points);
|
|
if (chain2_flip)
|
|
chain2->flip(end_points);
|
|
assert(chain1 == nullptr || chain1->begin == end_point1 || chain1->end == end_point1);
|
|
assert(chain2 == nullptr || chain2->begin == end_point2 || chain2->end == end_point2);
|
|
size_t chain_id = chains.merge(end_point1_chain_id, end_point2_chain_id);
|
|
Chain &chain = chains.chain(chain_id);
|
|
{
|
|
Chain chain_dst;
|
|
chain_dst.begin = (chain1 == nullptr) ? end_point1_other : (chain1->begin == end_point1) ? chain1->end : chain1->begin;
|
|
chain_dst.end = (chain2 == nullptr) ? end_point2_other : (chain2->begin == end_point2) ? chain2->end : chain2->begin;
|
|
chain_dst.cost = (chain1 == 0 ? 0. : chain1->cost) + (chain2 == 0 ? 0. : chain2->cost) + (end_point2->pos - end_point1->pos).norm();
|
|
chain_dst.cost_flipped = (chain1 == 0 ? 0. : chain1->cost_flipped) + (chain2 == 0 ? 0. : chain2->cost_flipped) + (end_point2_other->pos - end_point1_other->pos).norm();
|
|
chain_dst.num_segments = (chain1 == 0 ? 1 : chain1->num_segments) + (chain2 == 0 ? 1 : chain2->num_segments);
|
|
chain_dst.equivalent_with = chain_id;
|
|
chain = chain_dst;
|
|
}
|
|
if (chain.begin != end_point1_other && ! end_point1_other->heap_idx_invalid())
|
|
queue.remove(end_point1_other->heap_idx);
|
|
if (chain.end != end_point2_other && ! end_point2_other->heap_idx_invalid())
|
|
queue.remove(end_point2_other->heap_idx);
|
|
end_point1->edge_out = end_point2;
|
|
end_point2->edge_out = end_point1;
|
|
end_point1->chain_id = chain_id;
|
|
end_point2->chain_id = chain_id;
|
|
end_point1_other->chain_id = chain_id;
|
|
end_point2_other->chain_id = chain_id;
|
|
if (chain.begin != first_point)
|
|
chain.begin->chain_id = 0;
|
|
if (chain.end != first_point)
|
|
chain.end->chain_id = 0;
|
|
if (-- num_connections_to_end == 0) {
|
|
assert(validate_graph_and_queue());
|
|
// Last iteration. There shall be exactly one or two end points waiting to be connected.
|
|
assert(queue.size() <= ((first_point == nullptr) ? 4 : 2));
|
|
if (first_point == nullptr) {
|
|
// Find the first remaining end point.
|
|
do {
|
|
first_point = queue.top();
|
|
queue.pop();
|
|
} while (first_point->edge_out != nullptr);
|
|
assert(first_point->edge_out == nullptr);
|
|
}
|
|
// Find the first remaining end point.
|
|
do {
|
|
last_point = queue.top();
|
|
queue.pop();
|
|
} while (last_point->edge_out != nullptr);
|
|
assert(last_point->edge_out == nullptr);
|
|
#ifndef NDEBUG
|
|
while (! queue.empty()) {
|
|
assert(queue.top()->edge_out != nullptr && queue.top()->chain_id > 0);
|
|
queue.pop();
|
|
}
|
|
#endif /* NDEBUG */
|
|
break;
|
|
} else {
|
|
//FIXME update the 2nd end points on the queue.
|
|
// Update end points of the flipped segments.
|
|
update_end_point_in_queue(queue, kdtree, chains, end_points, chain.begin->opposite(end_points), first_point_idx, first_point);
|
|
update_end_point_in_queue(queue, kdtree, chains, end_points, chain.end->opposite(end_points), first_point_idx, first_point);
|
|
if (chain1_flip)
|
|
update_end_point_in_queue(queue, kdtree, chains, end_points, *chain.begin, first_point_idx, first_point);
|
|
if (chain2_flip)
|
|
update_end_point_in_queue(queue, kdtree, chains, end_points, *chain.end, first_point_idx, first_point);
|
|
// End points of chains shall certainly stay in the queue.
|
|
assert(chain.begin == first_point || chain.begin->heap_idx < queue.size());
|
|
assert(chain.end == first_point || chain.end ->heap_idx < queue.size());
|
|
assert(&chain.begin->opposite(end_points) != first_point &&
|
|
(chain.begin == first_point ? chain.begin->opposite(end_points).heap_idx_invalid() : chain.begin->opposite(end_points).heap_idx < queue.size()));
|
|
assert(&chain.end ->opposite(end_points) != first_point &&
|
|
(chain.end == first_point ? chain.end ->opposite(end_points).heap_idx_invalid() : chain.end ->opposite(end_points).heap_idx < queue.size()));
|
|
|
|
}
|
|
} else {
|
|
// This edge forms a loop. Update end_point1 and try another one.
|
|
update_end_point_in_queue(queue, kdtree, chains, end_points, *end_point1, first_point_idx, first_point);
|
|
#ifndef NDEBUG
|
|
// Each edge shall be longer than the last one removed from the queue.
|
|
//assert(end_point1->distance_out > distance_taken_last - SCALED_EPSILON);
|
|
if (end_point1->distance_out < distance_taken_last - SCALED_EPSILON) {
|
|
// printf("Warning: taking shorter length than previously is suspicious\n");
|
|
}
|
|
#endif /* NDEBUG */
|
|
//FIXME Remove the other end point from the KD tree.
|
|
// As the KD tree update is expensive, do it only after some larger number of points is removed from the queue.
|
|
}
|
|
assert(validate_graph_and_queue());
|
|
}
|
|
assert(queue.empty());
|
|
|
|
// Now interconnect pairs of segments into a chain.
|
|
assert(first_point != nullptr);
|
|
out.reserve(num_segments);
|
|
bool failed = false;
|
|
do {
|
|
assert(out.size() < num_segments);
|
|
size_t first_point_id = first_point - &end_points.front();
|
|
size_t segment_id = first_point_id >> 1;
|
|
bool reverse = (first_point_id & 1) != 0;
|
|
EndPoint *second_point = &end_points[first_point_id ^ 1];
|
|
if (REVERSE_COULD_FAIL) {
|
|
if (reverse && ! could_reverse_func(segment_id)) {
|
|
failed = true;
|
|
break;
|
|
}
|
|
} else {
|
|
assert(! reverse || could_reverse_func(segment_id));
|
|
}
|
|
out.emplace_back(segment_id, reverse);
|
|
first_point = second_point->edge_out;
|
|
} while (first_point != nullptr);
|
|
if (REVERSE_COULD_FAIL) {
|
|
if (failed) {
|
|
if (start_near == nullptr) {
|
|
// We may try the reverse order.
|
|
out.clear();
|
|
first_point = last_point;
|
|
failed = false;
|
|
do {
|
|
assert(out.size() < num_segments);
|
|
size_t first_point_id = first_point - &end_points.front();
|
|
size_t segment_id = first_point_id >> 1;
|
|
bool reverse = (first_point_id & 1) != 0;
|
|
EndPoint *second_point = &end_points[first_point_id ^ 1];
|
|
if (reverse && ! could_reverse_func(segment_id)) {
|
|
failed = true;
|
|
break;
|
|
}
|
|
out.emplace_back(segment_id, reverse);
|
|
first_point = second_point->edge_out;
|
|
} while (first_point != nullptr);
|
|
}
|
|
}
|
|
if (failed)
|
|
// As a last resort, try a dumb algorithm, which is not sensitive to edge reversal constraints.
|
|
out = chain_segments_closest_point<EndPoint, decltype(kdtree), CouldReverseFunc>(end_points, kdtree, could_reverse_func, (initial_point != nullptr) ? *initial_point : end_points.front());
|
|
} else {
|
|
assert(! failed);
|
|
}
|
|
}
|
|
|
|
assert(out.size() == num_segments);
|
|
return out;
|
|
}
|
|
|
|
template<typename PointType, typename SegmentEndPointFunc, typename CouldReverseFunc>
|
|
std::vector<std::pair<size_t, bool>> chain_segments_greedy_constrained_reversals(SegmentEndPointFunc end_point_func, CouldReverseFunc could_reverse_func, size_t num_segments, const PointType *start_near)
|
|
{
|
|
return chain_segments_greedy_constrained_reversals_<PointType, SegmentEndPointFunc, true, CouldReverseFunc>(end_point_func, could_reverse_func, num_segments, start_near);
|
|
}
|
|
|
|
template<typename PointType, typename SegmentEndPointFunc>
|
|
std::vector<std::pair<size_t, bool>> chain_segments_greedy(SegmentEndPointFunc end_point_func, size_t num_segments, const PointType *start_near)
|
|
{
|
|
auto could_reverse_func = [](size_t /* idx */) -> bool { return true; };
|
|
return chain_segments_greedy_constrained_reversals_<PointType, SegmentEndPointFunc, false, decltype(could_reverse_func)>(end_point_func, could_reverse_func, num_segments, start_near);
|
|
}
|
|
|
|
template<typename PointType, typename SegmentEndPointFunc, typename CouldReverseFunc>
|
|
std::vector<std::pair<size_t, bool>> chain_segments_greedy_constrained_reversals2(SegmentEndPointFunc end_point_func, CouldReverseFunc could_reverse_func, size_t num_segments, const PointType *start_near)
|
|
{
|
|
return chain_segments_greedy_constrained_reversals2_<PointType, SegmentEndPointFunc, true, CouldReverseFunc>(end_point_func, could_reverse_func, num_segments, start_near);
|
|
}
|
|
|
|
template<typename PointType, typename SegmentEndPointFunc>
|
|
std::vector<std::pair<size_t, bool>> chain_segments_greedy2(SegmentEndPointFunc end_point_func, size_t num_segments, const PointType *start_near)
|
|
{
|
|
auto could_reverse_func = [](size_t /* idx */) -> bool { return true; };
|
|
return chain_segments_greedy_constrained_reversals2_<PointType, SegmentEndPointFunc, false, decltype(could_reverse_func)>(end_point_func, could_reverse_func, num_segments, start_near);
|
|
}
|
|
|
|
std::vector<std::pair<size_t, bool>> chain_extrusion_entities(std::vector<ExtrusionEntity*> &entities, const Point *start_near)
|
|
{
|
|
auto segment_end_point = [&entities](size_t idx, bool first_point) -> const Point& { return first_point ? entities[idx]->first_point() : entities[idx]->last_point(); };
|
|
auto could_reverse = [&entities](size_t idx) { const ExtrusionEntity *ee = entities[idx]; return ee->is_loop() || ee->can_reverse(); };
|
|
std::vector<std::pair<size_t, bool>> out = chain_segments_greedy_constrained_reversals<Point, decltype(segment_end_point), decltype(could_reverse)>(segment_end_point, could_reverse, entities.size(), start_near);
|
|
for (std::pair<size_t, bool> &segment : out) {
|
|
ExtrusionEntity *ee = entities[segment.first];
|
|
if (ee->is_loop())
|
|
// Ignore reversals for loops, as the start point equals the end point.
|
|
segment.second = false;
|
|
// Is can_reverse() respected by the reversals?
|
|
assert(ee->can_reverse() || ! segment.second);
|
|
}
|
|
return out;
|
|
}
|
|
|
|
void reorder_extrusion_entities(std::vector<ExtrusionEntity*> &entities, const std::vector<std::pair<size_t, bool>> &chain)
|
|
{
|
|
assert(entities.size() == chain.size());
|
|
std::vector<ExtrusionEntity*> out;
|
|
out.reserve(entities.size());
|
|
for (const std::pair<size_t, bool> &idx : chain) {
|
|
assert(entities[idx.first] != nullptr);
|
|
out.emplace_back(entities[idx.first]);
|
|
if (idx.second)
|
|
out.back()->reverse();
|
|
}
|
|
entities.swap(out);
|
|
}
|
|
|
|
void chain_and_reorder_extrusion_entities(std::vector<ExtrusionEntity*> &entities, const Point *start_near)
|
|
{
|
|
reorder_extrusion_entities(entities, chain_extrusion_entities(entities, start_near));
|
|
}
|
|
|
|
std::vector<std::pair<size_t, bool>> chain_extrusion_paths(std::vector<ExtrusionPath> &extrusion_paths, const Point *start_near)
|
|
{
|
|
auto segment_end_point = [&extrusion_paths](size_t idx, bool first_point) -> const Point& { return first_point ? extrusion_paths[idx].first_point() : extrusion_paths[idx].last_point(); };
|
|
return chain_segments_greedy<Point, decltype(segment_end_point)>(segment_end_point, extrusion_paths.size(), start_near);
|
|
}
|
|
|
|
void reorder_extrusion_paths(std::vector<ExtrusionPath> &extrusion_paths, const std::vector<std::pair<size_t, bool>> &chain)
|
|
{
|
|
assert(extrusion_paths.size() == chain.size());
|
|
std::vector<ExtrusionPath> out;
|
|
out.reserve(extrusion_paths.size());
|
|
for (const std::pair<size_t, bool> &idx : chain) {
|
|
out.emplace_back(std::move(extrusion_paths[idx.first]));
|
|
if (idx.second)
|
|
out.back().reverse();
|
|
}
|
|
extrusion_paths.swap(out);
|
|
}
|
|
|
|
void chain_and_reorder_extrusion_paths(std::vector<ExtrusionPath> &extrusion_paths, const Point *start_near)
|
|
{
|
|
reorder_extrusion_paths(extrusion_paths, chain_extrusion_paths(extrusion_paths, start_near));
|
|
}
|
|
|
|
std::vector<size_t> chain_points(const Points &points, Point *start_near)
|
|
{
|
|
auto segment_end_point = [&points](size_t idx, bool /* first_point */) -> const Point& { return points[idx]; };
|
|
std::vector<std::pair<size_t, bool>> ordered = chain_segments_greedy<Point, decltype(segment_end_point)>(segment_end_point, points.size(), start_near);
|
|
std::vector<size_t> out;
|
|
out.reserve(ordered.size());
|
|
for (auto &segment_and_reversal : ordered)
|
|
out.emplace_back(segment_and_reversal.first);
|
|
return out;
|
|
}
|
|
|
|
#ifndef NDEBUG
|
|
// #define DEBUG_SVG_OUTPUT
|
|
#endif /* NDEBUG */
|
|
|
|
#ifdef DEBUG_SVG_OUTPUT
|
|
void svg_draw_polyline_chain(const char *name, size_t idx, const Polylines &polylines)
|
|
{
|
|
BoundingBox bbox = get_extents(polylines);
|
|
SVG svg(debug_out_path("%s-%d.svg", name, idx).c_str(), bbox);
|
|
svg.draw(polylines);
|
|
for (size_t i = 1; i < polylines.size(); ++i)
|
|
svg.draw(Line(polylines[i - 1].last_point(), polylines[i].first_point()), "red");
|
|
}
|
|
#endif /* DEBUG_SVG_OUTPUT */
|
|
|
|
#if 0
|
|
// Flip the sequences of polylines to lower the total length of connecting lines.
|
|
static inline void improve_ordering_by_segment_flipping(Polylines &polylines, bool fixed_start)
|
|
{
|
|
#ifndef NDEBUG
|
|
auto cost = [&polylines]() {
|
|
double sum = 0.;
|
|
for (size_t i = 1; i < polylines.size(); ++i)
|
|
sum += (polylines[i].first_point() - polylines[i - 1].last_point()).cast<double>().norm();
|
|
return sum;
|
|
};
|
|
double cost_initial = cost();
|
|
|
|
static int iRun = 0;
|
|
++ iRun;
|
|
#ifdef DEBUG_SVG_OUTPUT
|
|
svg_draw_polyline_chain("improve_ordering_by_segment_flipping-initial", iRun, polylines);
|
|
#endif /* DEBUG_SVG_OUTPUT */
|
|
#endif /* NDEBUG */
|
|
|
|
struct Connection {
|
|
Connection(size_t heap_idx = std::numeric_limits<size_t>::max(), bool flipped = false) : heap_idx(heap_idx), flipped(flipped) {}
|
|
// Position of this object on MutablePriorityHeap.
|
|
size_t heap_idx;
|
|
// Is segment_idx flipped?
|
|
bool flipped;
|
|
|
|
double squaredNorm(const Polylines &polylines, const std::vector<Connection> &connections) const
|
|
{ return ((this + 1)->start_point(polylines, connections) - this->end_point(polylines, connections)).squaredNorm(); }
|
|
double norm(const Polylines &polylines, const std::vector<Connection> &connections) const
|
|
{ return sqrt(this->squaredNorm(polylines, connections)); }
|
|
double squaredNorm(const Polylines &polylines, const std::vector<Connection> &connections, bool try_flip1, bool try_flip2) const
|
|
{ return ((this + 1)->start_point(polylines, connections, try_flip2) - this->end_point(polylines, connections, try_flip1)).squaredNorm(); }
|
|
double norm(const Polylines &polylines, const std::vector<Connection> &connections, bool try_flip1, bool try_flip2) const
|
|
{ return sqrt(this->squaredNorm(polylines, connections, try_flip1, try_flip2)); }
|
|
Vec2d start_point(const Polylines &polylines, const std::vector<Connection> &connections, bool flip = false) const
|
|
{ const Polyline &pl = polylines[this - connections.data()]; return ((this->flipped == flip) ? pl.points.front() : pl.points.back()).cast<double>(); }
|
|
Vec2d end_point(const Polylines &polylines, const std::vector<Connection> &connections, bool flip = false) const
|
|
{ const Polyline &pl = polylines[this - connections.data()]; return ((this->flipped == flip) ? pl.points.back() : pl.points.front()).cast<double>(); }
|
|
|
|
bool in_queue() const { return this->heap_idx != std::numeric_limits<size_t>::max(); }
|
|
void flip() { this->flipped = ! this->flipped; }
|
|
};
|
|
std::vector<Connection> connections(polylines.size());
|
|
|
|
#ifndef NDEBUG
|
|
auto cost_flipped = [fixed_start, &polylines, &connections]() {
|
|
assert(! fixed_start || ! connections.front().flipped);
|
|
double sum = 0.;
|
|
for (size_t i = 1; i < polylines.size(); ++ i)
|
|
sum += connections[i - 1].norm(polylines, connections);
|
|
return sum;
|
|
};
|
|
double cost_prev = cost_flipped();
|
|
assert(std::abs(cost_initial - cost_prev) < SCALED_EPSILON);
|
|
|
|
auto print_statistics = [&polylines, &connections]() {
|
|
#if 0
|
|
for (size_t i = 1; i < polylines.size(); ++ i)
|
|
printf("Connecting %d with %d: Current length %lf flip(%d, %d), left flipped: %lf, right flipped: %lf, both flipped: %lf, \n",
|
|
int(i - 1), int(i),
|
|
unscale<double>(connections[i - 1].norm(polylines, connections)),
|
|
int(connections[i - 1].flipped), int(connections[i].flipped),
|
|
unscale<double>(connections[i - 1].norm(polylines, connections, true, false)),
|
|
unscale<double>(connections[i - 1].norm(polylines, connections, false, true)),
|
|
unscale<double>(connections[i - 1].norm(polylines, connections, true, true)));
|
|
#endif
|
|
};
|
|
print_statistics();
|
|
#endif /* NDEBUG */
|
|
|
|
// Initialize a MutablePriorityHeap of connections between polylines.
|
|
auto queue = make_mutable_priority_queue<Connection*, false>(
|
|
[](Connection *connection, size_t idx){ connection->heap_idx = idx; },
|
|
// Sort by decreasing connection distance.
|
|
[&polylines, &connections](Connection *l, Connection *r){ return l->squaredNorm(polylines, connections) > r->squaredNorm(polylines, connections); });
|
|
queue.reserve(polylines.size() - 1);
|
|
for (size_t i = 0; i + 1 < polylines.size(); ++ i)
|
|
queue.push(&connections[i]);
|
|
|
|
static constexpr size_t itercnt = 100;
|
|
size_t iter = 0;
|
|
for (; ! queue.empty() && iter < itercnt; ++ iter) {
|
|
Connection &connection = *queue.top();
|
|
queue.pop();
|
|
connection.heap_idx = std::numeric_limits<size_t>::max();
|
|
size_t idx_first = &connection - connections.data();
|
|
// Try to flip segments starting with idx_first + 1 to the end.
|
|
// Calculate the last segment to be flipped to improve the total path length.
|
|
double length_current = connection.norm(polylines, connections);
|
|
double length_flipped = connection.norm(polylines, connections, false, true);
|
|
int best_idx_forward = int(idx_first);
|
|
double best_improvement_forward = 0.;
|
|
for (size_t i = idx_first + 1; i + 1 < connections.size(); ++ i) {
|
|
length_current += connections[i].norm(polylines, connections);
|
|
double this_improvement = length_current - length_flipped - connections[i].norm(polylines, connections, true, false);
|
|
length_flipped += connections[i].norm(polylines, connections, true, true);
|
|
if (this_improvement > best_improvement_forward) {
|
|
best_improvement_forward = this_improvement;
|
|
best_idx_forward = int(i);
|
|
}
|
|
// if (length_flipped > 1.5 * length_current)
|
|
// break;
|
|
}
|
|
if (length_current - length_flipped > best_improvement_forward)
|
|
// Best improvement by flipping up to the end.
|
|
best_idx_forward = int(connections.size()) - 1;
|
|
// Try to flip segments starting with idx_first - 1 to the start.
|
|
// Calculate the last segment to be flipped to improve the total path length.
|
|
length_current = connection.norm(polylines, connections);
|
|
length_flipped = connection.norm(polylines, connections, true, false);
|
|
int best_idx_backwards = int(idx_first);
|
|
double best_improvement_backwards = 0.;
|
|
for (int i = int(idx_first) - 1; i >= 0; -- i) {
|
|
length_current += connections[i].norm(polylines, connections);
|
|
double this_improvement = length_current - length_flipped - connections[i].norm(polylines, connections, false, true);
|
|
length_flipped += connections[i].norm(polylines, connections, true, true);
|
|
if (this_improvement > best_improvement_backwards) {
|
|
best_improvement_backwards = this_improvement;
|
|
best_idx_backwards = int(i);
|
|
}
|
|
// if (length_flipped > 1.5 * length_current)
|
|
// break;
|
|
}
|
|
if (! fixed_start && length_current - length_flipped > best_improvement_backwards)
|
|
// Best improvement by flipping up to the start including the first polyline.
|
|
best_idx_backwards = -1;
|
|
int update_begin = int(idx_first);
|
|
int update_end = best_idx_forward;
|
|
if (best_improvement_backwards > 0. && best_improvement_backwards > best_improvement_forward) {
|
|
// Flip the sequence of polylines from idx_first to best_improvement_forward + 1.
|
|
update_begin = best_idx_backwards;
|
|
update_end = int(idx_first);
|
|
}
|
|
assert(update_begin <= update_end);
|
|
if (update_begin == update_end)
|
|
continue;
|
|
for (int i = update_begin + 1; i <= update_end; ++ i)
|
|
connections[i].flip();
|
|
|
|
#ifndef NDEBUG
|
|
double cost = cost_flipped();
|
|
assert(cost < cost_prev);
|
|
cost_prev = cost;
|
|
print_statistics();
|
|
#endif /* NDEBUG */
|
|
|
|
update_end = std::min(update_end + 1, int(connections.size()) - 1);
|
|
for (int i = std::max(0, update_begin); i < update_end; ++ i) {
|
|
Connection &c = connections[i];
|
|
if (c.in_queue())
|
|
queue.update(c.heap_idx);
|
|
else
|
|
queue.push(&c);
|
|
}
|
|
}
|
|
|
|
// Flip the segments based on the flip flag.
|
|
for (Polyline &pl : polylines)
|
|
if (connections[&pl - polylines.data()].flipped)
|
|
pl.reverse();
|
|
|
|
#ifndef NDEBUG
|
|
double cost_final = cost();
|
|
#ifdef DEBUG_SVG_OUTPUT
|
|
svg_draw_polyline_chain("improve_ordering_by_segment_flipping-final", iRun, polylines);
|
|
#endif /* DEBUG_SVG_OUTPUT */
|
|
assert(cost_final <= cost_prev);
|
|
assert(cost_final <= cost_initial);
|
|
#endif /* NDEBUG */
|
|
}
|
|
#endif
|
|
|
|
struct FlipEdge {
|
|
FlipEdge(const Vec2d &p1, const Vec2d &p2, size_t source_index) : p1(p1), p2(p2), source_index(source_index) {}
|
|
void flip() { std::swap(this->p1, this->p2); }
|
|
Vec2d p1;
|
|
Vec2d p2;
|
|
size_t source_index;
|
|
};
|
|
|
|
struct ConnectionCost {
|
|
ConnectionCost(double cost, double cost_flipped) : cost(cost), cost_flipped(cost_flipped) {}
|
|
ConnectionCost() : cost(0.), cost_flipped(0.) {}
|
|
void flip() { std::swap(this->cost, this->cost_flipped); }
|
|
double cost = 0;
|
|
double cost_flipped = 0;
|
|
};
|
|
static inline ConnectionCost operator-(const ConnectionCost &lhs, const ConnectionCost& rhs) { return ConnectionCost(lhs.cost - rhs.cost, lhs.cost_flipped - rhs.cost_flipped); }
|
|
|
|
static inline std::pair<double, size_t> minimum_crossover_cost(
|
|
const std::vector<FlipEdge> &edges,
|
|
const std::pair<size_t, size_t> &span1, const ConnectionCost &cost1,
|
|
const std::pair<size_t, size_t> &span2, const ConnectionCost &cost2,
|
|
const std::pair<size_t, size_t> &span3, const ConnectionCost &cost3,
|
|
const double cost_current)
|
|
{
|
|
auto connection_cost = [&edges](
|
|
const std::pair<size_t, size_t> &span1, const ConnectionCost &cost1, bool reversed1, bool flipped1,
|
|
const std::pair<size_t, size_t> &span2, const ConnectionCost &cost2, bool reversed2, bool flipped2,
|
|
const std::pair<size_t, size_t> &span3, const ConnectionCost &cost3, bool reversed3, bool flipped3) {
|
|
auto first_point = [&edges](const std::pair<size_t, size_t> &span, bool flipped) { return flipped ? edges[span.first].p2 : edges[span.first].p1; };
|
|
auto last_point = [&edges](const std::pair<size_t, size_t> &span, bool flipped) { return flipped ? edges[span.second - 1].p1 : edges[span.second - 1].p2; };
|
|
auto point = [first_point, last_point](const std::pair<size_t, size_t> &span, bool start, bool flipped) { return start ? first_point(span, flipped) : last_point(span, flipped); };
|
|
auto cost = [](const ConnectionCost &acost, bool flipped) {
|
|
assert(acost.cost >= 0. && acost.cost_flipped >= 0.);
|
|
return flipped ? acost.cost_flipped : acost.cost;
|
|
};
|
|
// Ignore reversed single segment spans.
|
|
auto simple_span_ignore = [](const std::pair<size_t, size_t>& span, bool reversed) {
|
|
return span.first + 1 == span.second && reversed;
|
|
};
|
|
assert(span1.first < span1.second);
|
|
assert(span2.first < span2.second);
|
|
assert(span3.first < span3.second);
|
|
return
|
|
simple_span_ignore(span1, reversed1) || simple_span_ignore(span2, reversed2) || simple_span_ignore(span3, reversed3) ?
|
|
// Don't perform unnecessary calculations simulating reversion of single segment spans.
|
|
std::numeric_limits<double>::max() :
|
|
// Calculate the cost of reverting chains and / or flipping segment orientations.
|
|
cost(cost1, flipped1) + cost(cost2, flipped2) + cost(cost3, flipped3) +
|
|
(point(span2, ! reversed2, flipped2) - point(span1, reversed1, flipped1)).norm() +
|
|
(point(span3, ! reversed3, flipped3) - point(span2, reversed2, flipped2)).norm();
|
|
};
|
|
|
|
#ifndef NDEBUG
|
|
{
|
|
double c = connection_cost(span1, cost1, false, false, span2, cost2, false, false, span3, cost3, false, false);
|
|
assert(std::abs(c - cost_current) < SCALED_EPSILON);
|
|
}
|
|
#endif /* NDEBUG */
|
|
|
|
double cost_min = cost_current;
|
|
size_t flip_min = 0; // no flip, no improvement
|
|
for (size_t i = 0; i < (1 << 6); ++ i) {
|
|
// From the three combinations of 1,2,3 ordering, the other three are reversals of the first three.
|
|
double c1 = (i == 0) ? cost_current :
|
|
connection_cost(span1, cost1, (i & 1) != 0, (i & (1 << 1)) != 0, span2, cost2, (i & (1 << 2)) != 0, (i & (1 << 3)) != 0, span3, cost3, (i & (1 << 4)) != 0, (i & (1 << 5)) != 0);
|
|
double c2 = connection_cost(span1, cost1, (i & 1) != 0, (i & (1 << 1)) != 0, span3, cost3, (i & (1 << 2)) != 0, (i & (1 << 3)) != 0, span2, cost2, (i & (1 << 4)) != 0, (i & (1 << 5)) != 0);
|
|
double c3 = connection_cost(span2, cost2, (i & 1) != 0, (i & (1 << 1)) != 0, span1, cost1, (i & (1 << 2)) != 0, (i & (1 << 3)) != 0, span3, cost3, (i & (1 << 4)) != 0, (i & (1 << 5)) != 0);
|
|
if (c1 < cost_min) {
|
|
cost_min = c1;
|
|
flip_min = i;
|
|
}
|
|
if (c2 < cost_min) {
|
|
cost_min = c2;
|
|
flip_min = i + (1 << 6);
|
|
}
|
|
if (c3 < cost_min) {
|
|
cost_min = c3;
|
|
flip_min = i + (2 << 6);
|
|
}
|
|
}
|
|
return std::make_pair(cost_min, flip_min);
|
|
}
|
|
|
|
#if 0
|
|
static inline std::pair<double, size_t> minimum_crossover_cost(
|
|
const std::vector<FlipEdge> &edges,
|
|
const std::pair<size_t, size_t> &span1, const ConnectionCost &cost1,
|
|
const std::pair<size_t, size_t> &span2, const ConnectionCost &cost2,
|
|
const std::pair<size_t, size_t> &span3, const ConnectionCost &cost3,
|
|
const std::pair<size_t, size_t> &span4, const ConnectionCost &cost4,
|
|
const double cost_current)
|
|
{
|
|
auto connection_cost = [&edges](
|
|
const std::pair<size_t, size_t> &span1, const ConnectionCost &cost1, bool reversed1, bool flipped1,
|
|
const std::pair<size_t, size_t> &span2, const ConnectionCost &cost2, bool reversed2, bool flipped2,
|
|
const std::pair<size_t, size_t> &span3, const ConnectionCost &cost3, bool reversed3, bool flipped3,
|
|
const std::pair<size_t, size_t> &span4, const ConnectionCost &cost4, bool reversed4, bool flipped4) {
|
|
auto first_point = [&edges](const std::pair<size_t, size_t> &span, bool flipped) { return flipped ? edges[span.first].p2 : edges[span.first].p1; };
|
|
auto last_point = [&edges](const std::pair<size_t, size_t> &span, bool flipped) { return flipped ? edges[span.second - 1].p1 : edges[span.second - 1].p2; };
|
|
auto point = [first_point, last_point](const std::pair<size_t, size_t> &span, bool start, bool flipped) { return start ? first_point(span, flipped) : last_point(span, flipped); };
|
|
auto cost = [](const ConnectionCost &acost, bool flipped) {
|
|
assert(acost.cost >= 0. && acost.cost_flipped >= 0.);
|
|
return flipped ? acost.cost_flipped : acost.cost;
|
|
};
|
|
// Ignore reversed single segment spans.
|
|
auto simple_span_ignore = [](const std::pair<size_t, size_t>& span, bool reversed) {
|
|
return span.first + 1 == span.second && reversed;
|
|
};
|
|
assert(span1.first < span1.second);
|
|
assert(span2.first < span2.second);
|
|
assert(span3.first < span3.second);
|
|
assert(span4.first < span4.second);
|
|
return
|
|
simple_span_ignore(span1, reversed1) || simple_span_ignore(span2, reversed2) || simple_span_ignore(span3, reversed3) || simple_span_ignore(span4, reversed4) ?
|
|
// Don't perform unnecessary calculations simulating reversion of single segment spans.
|
|
std::numeric_limits<double>::max() :
|
|
// Calculate the cost of reverting chains and / or flipping segment orientations.
|
|
cost(cost1, flipped1) + cost(cost2, flipped2) + cost(cost3, flipped3) + cost(cost4, flipped4) +
|
|
(point(span2, ! reversed2, flipped2) - point(span1, reversed1, flipped1)).norm() +
|
|
(point(span3, ! reversed3, flipped3) - point(span2, reversed2, flipped2)).norm() +
|
|
(point(span4, ! reversed4, flipped4) - point(span3, reversed3, flipped3)).norm();
|
|
};
|
|
|
|
#ifndef NDEBUG
|
|
{
|
|
double c = connection_cost(span1, cost1, false, false, span2, cost2, false, false, span3, cost3, false, false, span4, cost4, false, false);
|
|
assert(std::abs(c - cost_current) < SCALED_EPSILON);
|
|
}
|
|
#endif /* NDEBUG */
|
|
|
|
double cost_min = cost_current;
|
|
size_t flip_min = 0; // no flip, no improvement
|
|
for (size_t i = 0; i < (1 << 8); ++ i) {
|
|
// From the three combinations of 1,2,3 ordering, the other three are reversals of the first three.
|
|
size_t permutation = 0;
|
|
for (double c : {
|
|
(i == 0) ? cost_current :
|
|
connection_cost(span1, cost1, (i & 1) != 0, (i & (1 << 1)) != 0, span2, cost2, (i & (1 << 2)) != 0, (i & (1 << 3)) != 0, span3, cost3, (i & (1 << 4)) != 0, (i & (1 << 5)) != 0, span4, cost4, (i & (1 << 6)) != 0, (i & (1 << 7)) != 0),
|
|
connection_cost(span1, cost1, (i & 1) != 0, (i & (1 << 1)) != 0, span2, cost2, (i & (1 << 2)) != 0, (i & (1 << 3)) != 0, span4, cost4, (i & (1 << 4)) != 0, (i & (1 << 5)) != 0, span3, cost3, (i & (1 << 6)) != 0, (i & (1 << 7)) != 0),
|
|
connection_cost(span1, cost1, (i & 1) != 0, (i & (1 << 1)) != 0, span3, cost3, (i & (1 << 2)) != 0, (i & (1 << 3)) != 0, span2, cost2, (i & (1 << 4)) != 0, (i & (1 << 5)) != 0, span4, cost4, (i & (1 << 6)) != 0, (i & (1 << 7)) != 0),
|
|
connection_cost(span1, cost1, (i & 1) != 0, (i & (1 << 1)) != 0, span3, cost3, (i & (1 << 2)) != 0, (i & (1 << 3)) != 0, span4, cost4, (i & (1 << 4)) != 0, (i & (1 << 5)) != 0, span2, cost2, (i & (1 << 6)) != 0, (i & (1 << 7)) != 0),
|
|
connection_cost(span1, cost1, (i & 1) != 0, (i & (1 << 1)) != 0, span4, cost4, (i & (1 << 2)) != 0, (i & (1 << 3)) != 0, span2, cost2, (i & (1 << 4)) != 0, (i & (1 << 5)) != 0, span3, cost3, (i & (1 << 6)) != 0, (i & (1 << 7)) != 0),
|
|
connection_cost(span1, cost1, (i & 1) != 0, (i & (1 << 1)) != 0, span4, cost4, (i & (1 << 2)) != 0, (i & (1 << 3)) != 0, span3, cost3, (i & (1 << 4)) != 0, (i & (1 << 5)) != 0, span2, cost2, (i & (1 << 6)) != 0, (i & (1 << 7)) != 0),
|
|
connection_cost(span2, cost2, (i & 1) != 0, (i & (1 << 1)) != 0, span1, cost1, (i & (1 << 2)) != 0, (i & (1 << 3)) != 0, span3, cost3, (i & (1 << 4)) != 0, (i & (1 << 5)) != 0, span4, cost4, (i & (1 << 6)) != 0, (i & (1 << 7)) != 0),
|
|
connection_cost(span2, cost2, (i & 1) != 0, (i & (1 << 1)) != 0, span1, cost1, (i & (1 << 2)) != 0, (i & (1 << 3)) != 0, span4, cost4, (i & (1 << 4)) != 0, (i & (1 << 5)) != 0, span3, cost3, (i & (1 << 6)) != 0, (i & (1 << 7)) != 0),
|
|
connection_cost(span2, cost2, (i & 1) != 0, (i & (1 << 1)) != 0, span3, cost3, (i & (1 << 2)) != 0, (i & (1 << 3)) != 0, span1, cost1, (i & (1 << 4)) != 0, (i & (1 << 5)) != 0, span4, cost4, (i & (1 << 6)) != 0, (i & (1 << 7)) != 0),
|
|
connection_cost(span2, cost2, (i & 1) != 0, (i & (1 << 1)) != 0, span4, cost4, (i & (1 << 2)) != 0, (i & (1 << 3)) != 0, span1, cost1, (i & (1 << 4)) != 0, (i & (1 << 5)) != 0, span3, cost3, (i & (1 << 6)) != 0, (i & (1 << 7)) != 0),
|
|
connection_cost(span3, cost3, (i & 1) != 0, (i & (1 << 1)) != 0, span1, cost1, (i & (1 << 2)) != 0, (i & (1 << 3)) != 0, span2, cost2, (i & (1 << 4)) != 0, (i & (1 << 5)) != 0, span4, cost4, (i & (1 << 6)) != 0, (i & (1 << 7)) != 0),
|
|
connection_cost(span3, cost3, (i & 1) != 0, (i & (1 << 1)) != 0, span2, cost2, (i & (1 << 2)) != 0, (i & (1 << 3)) != 0, span1, cost1, (i & (1 << 4)) != 0, (i & (1 << 5)) != 0, span4, cost4, (i & (1 << 6)) != 0, (i & (1 << 7)) != 0)
|
|
}) {
|
|
if (c < cost_min) {
|
|
cost_min = c;
|
|
flip_min = i + (permutation << 8);
|
|
}
|
|
++ permutation;
|
|
}
|
|
}
|
|
return std::make_pair(cost_min, flip_min);
|
|
}
|
|
#endif
|
|
|
|
static inline void do_crossover(const std::vector<FlipEdge> &edges_in, std::vector<FlipEdge> &edges_out,
|
|
const std::pair<size_t, size_t> &span1, const std::pair<size_t, size_t> &span2, const std::pair<size_t, size_t> &span3,
|
|
size_t i)
|
|
{
|
|
assert(edges_in.size() == edges_out.size());
|
|
auto do_it = [&edges_in, &edges_out](
|
|
const std::pair<size_t, size_t> &span1, bool reversed1, bool flipped1,
|
|
const std::pair<size_t, size_t> &span2, bool reversed2, bool flipped2,
|
|
const std::pair<size_t, size_t> &span3, bool reversed3, bool flipped3) {
|
|
auto it_edges_out = edges_out.begin();
|
|
auto copy_span = [&edges_in, &it_edges_out](std::pair<size_t, size_t> span, bool reversed, bool flipped) {
|
|
assert(span.first < span.second);
|
|
auto it = it_edges_out;
|
|
if (reversed)
|
|
std::reverse_copy(edges_in.begin() + span.first, edges_in.begin() + span.second, it_edges_out);
|
|
else
|
|
std::copy (edges_in.begin() + span.first, edges_in.begin() + span.second, it_edges_out);
|
|
it_edges_out += span.second - span.first;
|
|
if (reversed != flipped) {
|
|
for (; it != it_edges_out; ++ it)
|
|
it->flip();
|
|
}
|
|
};
|
|
copy_span(span1, reversed1, flipped1);
|
|
copy_span(span2, reversed2, flipped2);
|
|
copy_span(span3, reversed3, flipped3);
|
|
};
|
|
switch (i >> 6) {
|
|
case 0:
|
|
do_it(span1, (i & 1) != 0, (i & (1 << 1)) != 0, span2, (i & (1 << 2)) != 0, (i & (1 << 3)) != 0, span3, (i & (1 << 4)) != 0, (i & (1 << 5)) != 0);
|
|
break;
|
|
case 1:
|
|
do_it(span1, (i & 1) != 0, (i & (1 << 1)) != 0, span3, (i & (1 << 2)) != 0, (i & (1 << 3)) != 0, span2, (i & (1 << 4)) != 0, (i & (1 << 5)) != 0);
|
|
break;
|
|
default:
|
|
assert((i >> 6) == 2);
|
|
do_it(span2, (i & 1) != 0, (i & (1 << 1)) != 0, span1, (i & (1 << 2)) != 0, (i & (1 << 3)) != 0, span3, (i & (1 << 4)) != 0, (i & (1 << 5)) != 0);
|
|
}
|
|
assert(edges_in.size() == edges_out.size());
|
|
}
|
|
|
|
#if 0
|
|
static inline void do_crossover(const std::vector<FlipEdge> &edges_in, std::vector<FlipEdge> &edges_out,
|
|
const std::pair<size_t, size_t> &span1, const std::pair<size_t, size_t> &span2, const std::pair<size_t, size_t> &span3, const std::pair<size_t, size_t> &span4,
|
|
size_t i)
|
|
{
|
|
assert(edges_in.size() == edges_out.size());
|
|
auto do_it = [&edges_in, &edges_out](
|
|
const std::pair<size_t, size_t> &span1, bool reversed1, bool flipped1,
|
|
const std::pair<size_t, size_t> &span2, bool reversed2, bool flipped2,
|
|
const std::pair<size_t, size_t> &span3, bool reversed3, bool flipped3,
|
|
const std::pair<size_t, size_t> &span4, bool reversed4, bool flipped4) {
|
|
auto it_edges_out = edges_out.begin();
|
|
auto copy_span = [&edges_in, &it_edges_out](std::pair<size_t, size_t> span, bool reversed, bool flipped) {
|
|
assert(span.first < span.second);
|
|
auto it = it_edges_out;
|
|
if (reversed)
|
|
std::reverse_copy(edges_in.begin() + span.first, edges_in.begin() + span.second, it_edges_out);
|
|
else
|
|
std::copy (edges_in.begin() + span.first, edges_in.begin() + span.second, it_edges_out);
|
|
it_edges_out += span.second - span.first;
|
|
if (reversed != flipped) {
|
|
for (; it != it_edges_out; ++ it)
|
|
it->flip();
|
|
}
|
|
};
|
|
copy_span(span1, reversed1, flipped1);
|
|
copy_span(span2, reversed2, flipped2);
|
|
copy_span(span3, reversed3, flipped3);
|
|
copy_span(span4, reversed4, flipped4);
|
|
};
|
|
switch (i >> 8) {
|
|
case 0:
|
|
assert(i != 0); // otherwise it would be a no-op
|
|
do_it(span1, (i & 1) != 0, (i & (1 << 1)) != 0, span2, (i & (1 << 2)) != 0, (i & (1 << 3)) != 0, span3, (i & (1 << 4)) != 0, (i & (1 << 5)) != 0, span4, (i & (1 << 6)) != 0, (i & (1 << 7)) != 0);
|
|
break;
|
|
case 1:
|
|
do_it(span1, (i & 1) != 0, (i & (1 << 1)) != 0, span2, (i & (1 << 2)) != 0, (i & (1 << 3)) != 0, span4, (i & (1 << 4)) != 0, (i & (1 << 5)) != 0, span3, (i & (1 << 6)) != 0, (i & (1 << 7)) != 0);
|
|
break;
|
|
case 2:
|
|
do_it(span1, (i & 1) != 0, (i & (1 << 1)) != 0, span3, (i & (1 << 2)) != 0, (i & (1 << 3)) != 0, span2, (i & (1 << 4)) != 0, (i & (1 << 5)) != 0, span4, (i & (1 << 6)) != 0, (i & (1 << 7)) != 0);
|
|
break;
|
|
case 3:
|
|
do_it(span1, (i & 1) != 0, (i & (1 << 1)) != 0, span3, (i & (1 << 2)) != 0, (i & (1 << 3)) != 0, span4, (i & (1 << 4)) != 0, (i & (1 << 5)) != 0, span2, (i & (1 << 6)) != 0, (i & (1 << 7)) != 0);
|
|
break;
|
|
case 4:
|
|
do_it(span1, (i & 1) != 0, (i & (1 << 1)) != 0, span4, (i & (1 << 2)) != 0, (i & (1 << 3)) != 0, span2, (i & (1 << 4)) != 0, (i & (1 << 5)) != 0, span3, (i & (1 << 6)) != 0, (i & (1 << 7)) != 0);
|
|
break;
|
|
case 5:
|
|
do_it(span1, (i & 1) != 0, (i & (1 << 1)) != 0, span4, (i & (1 << 2)) != 0, (i & (1 << 3)) != 0, span3, (i & (1 << 4)) != 0, (i & (1 << 5)) != 0, span2, (i & (1 << 6)) != 0, (i & (1 << 7)) != 0);
|
|
break;
|
|
case 6:
|
|
do_it(span2, (i & 1) != 0, (i & (1 << 1)) != 0, span1, (i & (1 << 2)) != 0, (i & (1 << 3)) != 0, span3, (i & (1 << 4)) != 0, (i & (1 << 5)) != 0, span4, (i & (1 << 6)) != 0, (i & (1 << 7)) != 0);
|
|
break;
|
|
case 7:
|
|
do_it(span2, (i & 1) != 0, (i & (1 << 1)) != 0, span1, (i & (1 << 2)) != 0, (i & (1 << 3)) != 0, span4, (i & (1 << 4)) != 0, (i & (1 << 5)) != 0, span3, (i & (1 << 6)) != 0, (i & (1 << 7)) != 0);
|
|
break;
|
|
case 8:
|
|
do_it(span2, (i & 1) != 0, (i & (1 << 1)) != 0, span3, (i & (1 << 2)) != 0, (i & (1 << 3)) != 0, span1, (i & (1 << 4)) != 0, (i & (1 << 5)) != 0, span4, (i & (1 << 6)) != 0, (i & (1 << 7)) != 0);
|
|
break;
|
|
case 9:
|
|
do_it(span2, (i & 1) != 0, (i & (1 << 1)) != 0, span4, (i & (1 << 2)) != 0, (i & (1 << 3)) != 0, span1, (i & (1 << 4)) != 0, (i & (1 << 5)) != 0, span3, (i & (1 << 6)) != 0, (i & (1 << 7)) != 0);
|
|
break;
|
|
case 10:
|
|
do_it(span3, (i & 1) != 0, (i & (1 << 1)) != 0, span1, (i & (1 << 2)) != 0, (i & (1 << 3)) != 0, span2, (i & (1 << 4)) != 0, (i & (1 << 5)) != 0, span4, (i & (1 << 6)) != 0, (i & (1 << 7)) != 0);
|
|
break;
|
|
default:
|
|
assert((i >> 8) == 11);
|
|
do_it(span3, (i & 1) != 0, (i & (1 << 1)) != 0, span2, (i & (1 << 2)) != 0, (i & (1 << 3)) != 0, span1, (i & (1 << 4)) != 0, (i & (1 << 5)) != 0, span4, (i & (1 << 6)) != 0, (i & (1 << 7)) != 0);
|
|
break;
|
|
}
|
|
assert(edges_in.size() == edges_out.size());
|
|
}
|
|
#endif
|
|
|
|
// Worst time complexity: O(min(n, 100) * (n * log n + n^2)
|
|
// Expected time complexity: O(min(n, 100) * (n * log n + k * n)
|
|
// where n is the number of edges and k is the number of connection_lengths candidates after the first one
|
|
// is found that improves the total cost.
|
|
//FIXME there are likley better heuristics to lower the time complexity.
|
|
static inline void reorder_by_two_exchanges_with_segment_flipping(std::vector<FlipEdge> &edges)
|
|
{
|
|
if (edges.size() < 2)
|
|
return;
|
|
|
|
std::vector<ConnectionCost> connections(edges.size());
|
|
std::vector<FlipEdge> edges_tmp(edges);
|
|
std::vector<std::pair<double, size_t>> connection_lengths(edges.size() - 1, std::pair<double, size_t>(0., 0));
|
|
std::vector<char> connection_tried(edges.size(), false);
|
|
const size_t max_iterations = std::min(edges.size(), size_t(100));
|
|
for (size_t iter = 0; iter < max_iterations; ++ iter) {
|
|
// Initialize connection costs and connection lengths.
|
|
for (size_t i = 1; i < edges.size(); ++ i) {
|
|
const FlipEdge &e1 = edges[i - 1];
|
|
const FlipEdge &e2 = edges[i];
|
|
ConnectionCost &c = connections[i];
|
|
c = connections[i - 1];
|
|
double l = (e2.p1 - e1.p2).norm();
|
|
c.cost += l;
|
|
c.cost_flipped += (e2.p2 - e1.p1).norm();
|
|
connection_lengths[i - 1] = std::make_pair(l, i);
|
|
}
|
|
std::sort(connection_lengths.begin(), connection_lengths.end(), [](const std::pair<double, size_t> &l, const std::pair<double, size_t> &r) { return l.first > r.first; });
|
|
std::fill(connection_tried.begin(), connection_tried.end(), false);
|
|
size_t crossover1_pos_final = std::numeric_limits<size_t>::max();
|
|
size_t crossover2_pos_final = std::numeric_limits<size_t>::max();
|
|
size_t crossover_flip_final = 0;
|
|
for (const std::pair<double, size_t>& first_crossover_candidate : connection_lengths) {
|
|
size_t longest_connection_idx = first_crossover_candidate.second;
|
|
connection_tried[longest_connection_idx] = true;
|
|
// Find the second crossover connection with the lowest total chain cost.
|
|
size_t crossover_pos_min = std::numeric_limits<size_t>::max();
|
|
double crossover_cost_min = connections.back().cost;
|
|
size_t crossover_flip_min = 0;
|
|
for (size_t j = 1; j < connections.size(); ++ j)
|
|
if (! connection_tried[j]) {
|
|
size_t a = j;
|
|
size_t b = longest_connection_idx;
|
|
if (a > b)
|
|
std::swap(a, b);
|
|
std::pair<double, size_t> cost_and_flip = minimum_crossover_cost(edges,
|
|
std::make_pair(size_t(0), a), connections[a - 1], std::make_pair(a, b), connections[b - 1] - connections[a], std::make_pair(b, edges.size()), connections.back() - connections[b],
|
|
connections.back().cost);
|
|
if (cost_and_flip.second > 0 && cost_and_flip.first < crossover_cost_min) {
|
|
crossover_pos_min = j;
|
|
crossover_cost_min = cost_and_flip.first;
|
|
crossover_flip_min = cost_and_flip.second;
|
|
assert(crossover_cost_min < connections.back().cost + EPSILON);
|
|
}
|
|
}
|
|
if (crossover_cost_min < connections.back().cost) {
|
|
// The cost of the chain with the proposed two crossovers has a lower total cost than the current chain. Apply the crossover.
|
|
crossover1_pos_final = longest_connection_idx;
|
|
crossover2_pos_final = crossover_pos_min;
|
|
crossover_flip_final = crossover_flip_min;
|
|
break;
|
|
} else {
|
|
// Continue with another long candidate edge.
|
|
}
|
|
}
|
|
if (crossover_flip_final > 0) {
|
|
// Pair of cross over positions and flip / reverse constellation has been found, which improves the total cost of the connection.
|
|
// Perform a crossover.
|
|
if (crossover1_pos_final > crossover2_pos_final)
|
|
std::swap(crossover1_pos_final, crossover2_pos_final);
|
|
do_crossover(edges, edges_tmp, std::make_pair(size_t(0), crossover1_pos_final), std::make_pair(crossover1_pos_final, crossover2_pos_final), std::make_pair(crossover2_pos_final, edges.size()), crossover_flip_final);
|
|
edges.swap(edges_tmp);
|
|
} else {
|
|
// No valid pair of cross over positions was found improving the total cost. Giving up.
|
|
break;
|
|
}
|
|
}
|
|
}
|
|
|
|
#if 0
|
|
// Currently not used, too slow.
|
|
static inline void reorder_by_three_exchanges_with_segment_flipping(std::vector<FlipEdge> &edges)
|
|
{
|
|
if (edges.size() < 3) {
|
|
reorder_by_two_exchanges_with_segment_flipping(edges);
|
|
return;
|
|
}
|
|
|
|
std::vector<ConnectionCost> connections(edges.size());
|
|
std::vector<FlipEdge> edges_tmp(edges);
|
|
std::vector<std::pair<double, size_t>> connection_lengths(edges.size() - 1, std::pair<double, size_t>(0., 0));
|
|
std::vector<char> connection_tried(edges.size(), false);
|
|
for (size_t iter = 0; iter < edges.size(); ++ iter) {
|
|
// Initialize connection costs and connection lengths.
|
|
for (size_t i = 1; i < edges.size(); ++ i) {
|
|
const FlipEdge &e1 = edges[i - 1];
|
|
const FlipEdge &e2 = edges[i];
|
|
ConnectionCost &c = connections[i];
|
|
c = connections[i - 1];
|
|
double l = (e2.p1 - e1.p2).norm();
|
|
c.cost += l;
|
|
c.cost_flipped += (e2.p2 - e1.p1).norm();
|
|
connection_lengths[i - 1] = std::make_pair(l, i);
|
|
}
|
|
std::sort(connection_lengths.begin(), connection_lengths.end(), [](const std::pair<double, size_t> &l, const std::pair<double, size_t> &r) { return l.first > r.first; });
|
|
std::fill(connection_tried.begin(), connection_tried.end(), false);
|
|
size_t crossover1_pos_final = std::numeric_limits<size_t>::max();
|
|
size_t crossover2_pos_final = std::numeric_limits<size_t>::max();
|
|
size_t crossover3_pos_final = std::numeric_limits<size_t>::max();
|
|
size_t crossover_flip_final = 0;
|
|
for (const std::pair<double, size_t> &first_crossover_candidate : connection_lengths) {
|
|
size_t longest_connection_idx = first_crossover_candidate.second;
|
|
connection_tried[longest_connection_idx] = true;
|
|
// Find the second crossover connection with the lowest total chain cost.
|
|
double crossover_cost_min = connections.back().cost;
|
|
for (size_t j = 1; j < connections.size(); ++ j)
|
|
if (! connection_tried[j]) {
|
|
for (size_t k = j + 1; k < connections.size(); ++ k)
|
|
if (! connection_tried[k]) {
|
|
size_t a = longest_connection_idx;
|
|
size_t b = j;
|
|
size_t c = k;
|
|
if (a > c)
|
|
std::swap(a, c);
|
|
if (a > b)
|
|
std::swap(a, b);
|
|
if (b > c)
|
|
std::swap(b, c);
|
|
std::pair<double, size_t> cost_and_flip = minimum_crossover_cost(edges,
|
|
std::make_pair(size_t(0), a), connections[a - 1], std::make_pair(a, b), connections[b - 1] - connections[a],
|
|
std::make_pair(b, c), connections[c - 1] - connections[b], std::make_pair(c, edges.size()), connections.back() - connections[c],
|
|
connections.back().cost);
|
|
if (cost_and_flip.second > 0 && cost_and_flip.first < crossover_cost_min) {
|
|
crossover_cost_min = cost_and_flip.first;
|
|
crossover1_pos_final = a;
|
|
crossover2_pos_final = b;
|
|
crossover3_pos_final = c;
|
|
crossover_flip_final = cost_and_flip.second;
|
|
assert(crossover_cost_min < connections.back().cost + EPSILON);
|
|
}
|
|
}
|
|
}
|
|
if (crossover_flip_final > 0) {
|
|
// The cost of the chain with the proposed two crossovers has a lower total cost than the current chain. Apply the crossover.
|
|
break;
|
|
} else {
|
|
// Continue with another long candidate edge.
|
|
}
|
|
}
|
|
if (crossover_flip_final > 0) {
|
|
// Pair of cross over positions and flip / reverse constellation has been found, which improves the total cost of the connection.
|
|
// Perform a crossover.
|
|
do_crossover(edges, edges_tmp, std::make_pair(size_t(0), crossover1_pos_final), std::make_pair(crossover1_pos_final, crossover2_pos_final),
|
|
std::make_pair(crossover2_pos_final, crossover3_pos_final), std::make_pair(crossover3_pos_final, edges.size()), crossover_flip_final);
|
|
edges.swap(edges_tmp);
|
|
} else {
|
|
// No valid pair of cross over positions was found improving the total cost. Giving up.
|
|
break;
|
|
}
|
|
}
|
|
}
|
|
#endif
|
|
|
|
typedef Eigen::Matrix<double, Eigen::Dynamic, Eigen::Dynamic, Eigen::DontAlign> Matrixd;
|
|
|
|
class FourOptCosts {
|
|
public:
|
|
FourOptCosts(const ConnectionCost &c1, const ConnectionCost &c2, const ConnectionCost &c3, const ConnectionCost &c4) : costs { &c1, &c2, &c3, &c4 } {}
|
|
|
|
double operator()(size_t piece_idx, bool flipped) const { return flipped ? costs[piece_idx]->cost_flipped : costs[piece_idx]->cost; }
|
|
|
|
private:
|
|
const ConnectionCost* costs[4];
|
|
};
|
|
|
|
#if 0
|
|
static inline std::pair<double, size_t> minimum_crossover_cost(
|
|
const FourOptCosts &segment_costs,
|
|
const Matrixd &segment_end_point_distance_matrix,
|
|
const double cost_current)
|
|
{
|
|
// Distance from the end of span1 to the start of span2.
|
|
auto end_point_distance = [&segment_end_point_distance_matrix](size_t span1, bool reversed1, bool flipped1, size_t span2, bool reversed2, bool flipped2) {
|
|
return segment_end_point_distance_matrix(span1 * 4 + (! reversed1) * 2 + flipped1, span2 * 4 + reversed2 * 2 + flipped2);
|
|
};
|
|
auto connection_cost = [&segment_costs, end_point_distance](
|
|
const size_t span1, bool reversed1, bool flipped1,
|
|
const size_t span2, bool reversed2, bool flipped2,
|
|
const size_t span3, bool reversed3, bool flipped3,
|
|
const size_t span4, bool reversed4, bool flipped4) {
|
|
// Calculate the cost of reverting chains and / or flipping segment orientations.
|
|
return segment_costs(span1, flipped1) + segment_costs(span2, flipped2) + segment_costs(span3, flipped3) + segment_costs(span4, flipped4) +
|
|
end_point_distance(span1, reversed1, flipped1, span2, reversed2, flipped2) +
|
|
end_point_distance(span2, reversed2, flipped2, span3, reversed3, flipped3) +
|
|
end_point_distance(span3, reversed3, flipped3, span4, reversed4, flipped4);
|
|
};
|
|
|
|
#ifndef NDEBUG
|
|
{
|
|
double c = connection_cost(0, false, false, 1, false, false, 2, false, false, 3, false, false);
|
|
assert(std::abs(c - cost_current) < SCALED_EPSILON);
|
|
}
|
|
#endif /* NDEBUG */
|
|
|
|
double cost_min = cost_current;
|
|
size_t flip_min = 0; // no flip, no improvement
|
|
for (size_t i = 0; i < (1 << 8); ++ i) {
|
|
// From the three combinations of 1,2,3 ordering, the other three are reversals of the first three.
|
|
size_t permutation = 0;
|
|
for (double c : {
|
|
(i == 0) ? cost_current :
|
|
connection_cost(0, (i & 1) != 0, (i & (1 << 1)) != 0, 1, (i & (1 << 2)) != 0, (i & (1 << 3)) != 0, 2, (i & (1 << 4)) != 0, (i & (1 << 5)) != 0, 3, (i & (1 << 6)) != 0, (i & (1 << 7)) != 0),
|
|
connection_cost(0, (i & 1) != 0, (i & (1 << 1)) != 0, 1, (i & (1 << 2)) != 0, (i & (1 << 3)) != 0, 3, (i & (1 << 4)) != 0, (i & (1 << 5)) != 0, 2, (i & (1 << 6)) != 0, (i & (1 << 7)) != 0),
|
|
connection_cost(0, (i & 1) != 0, (i & (1 << 1)) != 0, 2, (i & (1 << 2)) != 0, (i & (1 << 3)) != 0, 1, (i & (1 << 4)) != 0, (i & (1 << 5)) != 0, 3, (i & (1 << 6)) != 0, (i & (1 << 7)) != 0),
|
|
connection_cost(0, (i & 1) != 0, (i & (1 << 1)) != 0, 2, (i & (1 << 2)) != 0, (i & (1 << 3)) != 0, 3, (i & (1 << 4)) != 0, (i & (1 << 5)) != 0, 1, (i & (1 << 6)) != 0, (i & (1 << 7)) != 0),
|
|
connection_cost(0, (i & 1) != 0, (i & (1 << 1)) != 0, 3, (i & (1 << 2)) != 0, (i & (1 << 3)) != 0, 1, (i & (1 << 4)) != 0, (i & (1 << 5)) != 0, 2, (i & (1 << 6)) != 0, (i & (1 << 7)) != 0),
|
|
connection_cost(0, (i & 1) != 0, (i & (1 << 1)) != 0, 3, (i & (1 << 2)) != 0, (i & (1 << 3)) != 0, 2, (i & (1 << 4)) != 0, (i & (1 << 5)) != 0, 1, (i & (1 << 6)) != 0, (i & (1 << 7)) != 0),
|
|
connection_cost(1, (i & 1) != 0, (i & (1 << 1)) != 0, 0, (i & (1 << 2)) != 0, (i & (1 << 3)) != 0, 2, (i & (1 << 4)) != 0, (i & (1 << 5)) != 0, 3, (i & (1 << 6)) != 0, (i & (1 << 7)) != 0),
|
|
connection_cost(1, (i & 1) != 0, (i & (1 << 1)) != 0, 0, (i & (1 << 2)) != 0, (i & (1 << 3)) != 0, 3, (i & (1 << 4)) != 0, (i & (1 << 5)) != 0, 2, (i & (1 << 6)) != 0, (i & (1 << 7)) != 0),
|
|
connection_cost(1, (i & 1) != 0, (i & (1 << 1)) != 0, 2, (i & (1 << 2)) != 0, (i & (1 << 3)) != 0, 0, (i & (1 << 4)) != 0, (i & (1 << 5)) != 0, 3, (i & (1 << 6)) != 0, (i & (1 << 7)) != 0),
|
|
connection_cost(1, (i & 1) != 0, (i & (1 << 1)) != 0, 3, (i & (1 << 2)) != 0, (i & (1 << 3)) != 0, 0, (i & (1 << 4)) != 0, (i & (1 << 5)) != 0, 2, (i & (1 << 6)) != 0, (i & (1 << 7)) != 0),
|
|
connection_cost(2, (i & 1) != 0, (i & (1 << 1)) != 0, 0, (i & (1 << 2)) != 0, (i & (1 << 3)) != 0, 1, (i & (1 << 4)) != 0, (i & (1 << 5)) != 0, 3, (i & (1 << 6)) != 0, (i & (1 << 7)) != 0),
|
|
connection_cost(2, (i & 1) != 0, (i & (1 << 1)) != 0, 1, (i & (1 << 2)) != 0, (i & (1 << 3)) != 0, 0, (i & (1 << 4)) != 0, (i & (1 << 5)) != 0, 3, (i & (1 << 6)) != 0, (i & (1 << 7)) != 0)
|
|
}) {
|
|
if (c < cost_min) {
|
|
cost_min = c;
|
|
flip_min = i + (permutation << 8);
|
|
}
|
|
++ permutation;
|
|
}
|
|
}
|
|
return std::make_pair(cost_min, flip_min);
|
|
}
|
|
|
|
// Currently not used, too slow.
|
|
static inline void reorder_by_three_exchanges_with_segment_flipping2(std::vector<FlipEdge> &edges)
|
|
{
|
|
if (edges.size() < 3) {
|
|
reorder_by_two_exchanges_with_segment_flipping(edges);
|
|
return;
|
|
}
|
|
|
|
std::vector<ConnectionCost> connections(edges.size());
|
|
std::vector<FlipEdge> edges_tmp(edges);
|
|
std::vector<std::pair<double, size_t>> connection_lengths(edges.size() - 1, std::pair<double, size_t>(0., 0));
|
|
std::vector<char> connection_tried(edges.size(), false);
|
|
for (size_t iter = 0; iter < edges.size(); ++ iter) {
|
|
// Initialize connection costs and connection lengths.
|
|
for (size_t i = 1; i < edges.size(); ++ i) {
|
|
const FlipEdge &e1 = edges[i - 1];
|
|
const FlipEdge &e2 = edges[i];
|
|
ConnectionCost &c = connections[i];
|
|
c = connections[i - 1];
|
|
double l = (e2.p1 - e1.p2).norm();
|
|
c.cost += l;
|
|
c.cost_flipped += (e2.p2 - e1.p1).norm();
|
|
connection_lengths[i - 1] = std::make_pair(l, i);
|
|
}
|
|
std::sort(connection_lengths.begin(), connection_lengths.end(), [](const std::pair<double, size_t> &l, const std::pair<double, size_t> &r) { return l.first > r.first; });
|
|
std::fill(connection_tried.begin(), connection_tried.end(), false);
|
|
size_t crossover1_pos_final = std::numeric_limits<size_t>::max();
|
|
size_t crossover2_pos_final = std::numeric_limits<size_t>::max();
|
|
size_t crossover3_pos_final = std::numeric_limits<size_t>::max();
|
|
size_t crossover_flip_final = 0;
|
|
// Distances between the end points of the four pieces of the current segment sequence.
|
|
#ifdef NDEBUG
|
|
Matrixd segment_end_point_distance_matrix(4 * 4, 4 * 4);
|
|
#else /* NDEBUG */
|
|
Matrixd segment_end_point_distance_matrix = Matrixd::Constant(4 * 4, 4 * 4, std::numeric_limits<double>::max());
|
|
#endif /* NDEBUG */
|
|
for (const std::pair<double, size_t> &first_crossover_candidate : connection_lengths) {
|
|
size_t longest_connection_idx = first_crossover_candidate.second;
|
|
connection_tried[longest_connection_idx] = true;
|
|
// Find the second crossover connection with the lowest total chain cost.
|
|
double crossover_cost_min = connections.back().cost;
|
|
for (size_t j = 1; j < connections.size(); ++ j)
|
|
if (! connection_tried[j]) {
|
|
for (size_t k = j + 1; k < connections.size(); ++ k)
|
|
if (! connection_tried[k]) {
|
|
size_t a = longest_connection_idx;
|
|
size_t b = j;
|
|
size_t c = k;
|
|
if (a > c)
|
|
std::swap(a, c);
|
|
if (a > b)
|
|
std::swap(a, b);
|
|
if (b > c)
|
|
std::swap(b, c);
|
|
const Vec2d* endpts[16] = {
|
|
&edges[0].p1, &edges[0].p2, &edges[a - 1].p2, &edges[a - 1].p1,
|
|
&edges[a].p1, &edges[a].p2, &edges[b - 1].p2, &edges[b - 1].p1,
|
|
&edges[b].p1, &edges[b].p2, &edges[c - 1].p2, &edges[c - 1].p1,
|
|
&edges[c].p1, &edges[c].p2, &edges.back().p2, &edges.back().p1 };
|
|
for (size_t v = 0; v < 16; ++ v) {
|
|
const Vec2d &p1 = *endpts[v];
|
|
for (size_t u = (v & (~3)) + 4; u < 16; ++ u)
|
|
segment_end_point_distance_matrix(u, v) = segment_end_point_distance_matrix(v, u) = (*endpts[u] - p1).norm();
|
|
}
|
|
FourOptCosts segment_costs(connections[a - 1], connections[b - 1] - connections[a], connections[c - 1] - connections[b], connections.back() - connections[c]);
|
|
std::pair<double, size_t> cost_and_flip = minimum_crossover_cost(segment_costs, segment_end_point_distance_matrix, connections.back().cost);
|
|
if (cost_and_flip.second > 0 && cost_and_flip.first < crossover_cost_min) {
|
|
crossover_cost_min = cost_and_flip.first;
|
|
crossover1_pos_final = a;
|
|
crossover2_pos_final = b;
|
|
crossover3_pos_final = c;
|
|
crossover_flip_final = cost_and_flip.second;
|
|
assert(crossover_cost_min < connections.back().cost + EPSILON);
|
|
}
|
|
}
|
|
}
|
|
if (crossover_flip_final > 0) {
|
|
// The cost of the chain with the proposed two crossovers has a lower total cost than the current chain. Apply the crossover.
|
|
break;
|
|
} else {
|
|
// Continue with another long candidate edge.
|
|
}
|
|
}
|
|
if (crossover_flip_final > 0) {
|
|
// Pair of cross over positions and flip / reverse constellation has been found, which improves the total cost of the connection.
|
|
// Perform a crossover.
|
|
do_crossover(edges, edges_tmp, std::make_pair(size_t(0), crossover1_pos_final), std::make_pair(crossover1_pos_final, crossover2_pos_final),
|
|
std::make_pair(crossover2_pos_final, crossover3_pos_final), std::make_pair(crossover3_pos_final, edges.size()), crossover_flip_final);
|
|
edges.swap(edges_tmp);
|
|
} else {
|
|
// No valid pair of cross over positions was found improving the total cost. Giving up.
|
|
break;
|
|
}
|
|
}
|
|
}
|
|
#endif
|
|
|
|
// Flip the sequences of polylines to lower the total length of connecting lines.
|
|
// Used by the infill generator if the infill is not connected with perimeter lines
|
|
// and to order the brim lines.
|
|
static inline void improve_ordering_by_two_exchanges_with_segment_flipping(Polylines &polylines, bool fixed_start)
|
|
{
|
|
#ifndef NDEBUG
|
|
auto cost = [&polylines]() {
|
|
double sum = 0.;
|
|
for (size_t i = 1; i < polylines.size(); ++i)
|
|
sum += (polylines[i].first_point() - polylines[i - 1].last_point()).cast<double>().norm();
|
|
return sum;
|
|
};
|
|
double cost_initial = cost();
|
|
|
|
static int iRun = 0;
|
|
++ iRun;
|
|
#ifdef DEBUG_SVG_OUTPUT
|
|
svg_draw_polyline_chain("improve_ordering_by_two_exchanges_with_segment_flipping-initial", iRun, polylines);
|
|
#endif /* DEBUG_SVG_OUTPUT */
|
|
#endif /* NDEBUG */
|
|
|
|
std::vector<FlipEdge> edges;
|
|
edges.reserve(polylines.size());
|
|
std::transform(polylines.begin(), polylines.end(), std::back_inserter(edges),
|
|
[&polylines](const Polyline &pl){ return FlipEdge(pl.first_point().cast<double>(), pl.last_point().cast<double>(), &pl - polylines.data()); });
|
|
#if 1
|
|
reorder_by_two_exchanges_with_segment_flipping(edges);
|
|
#else
|
|
// reorder_by_three_exchanges_with_segment_flipping(edges);
|
|
reorder_by_three_exchanges_with_segment_flipping2(edges);
|
|
#endif
|
|
Polylines out;
|
|
out.reserve(polylines.size());
|
|
for (const FlipEdge &edge : edges) {
|
|
Polyline &pl = polylines[edge.source_index];
|
|
out.emplace_back(std::move(pl));
|
|
if (edge.p2 == pl.first_point().cast<double>()) {
|
|
// Polyline is flipped.
|
|
out.back().reverse();
|
|
} else {
|
|
// Polyline is not flipped.
|
|
assert(edge.p1 == pl.first_point().cast<double>());
|
|
}
|
|
}
|
|
|
|
#ifndef NDEBUG
|
|
double cost_final = cost();
|
|
#ifdef DEBUG_SVG_OUTPUT
|
|
svg_draw_polyline_chain("improve_ordering_by_two_exchanges_with_segment_flipping-final", iRun, out);
|
|
#endif /* DEBUG_SVG_OUTPUT */
|
|
assert(cost_final <= cost_initial);
|
|
#endif /* NDEBUG */
|
|
}
|
|
|
|
// Used to optimize order of infill lines and brim lines.
|
|
Polylines chain_polylines(Polylines &&polylines, const Point *start_near)
|
|
{
|
|
#ifdef DEBUG_SVG_OUTPUT
|
|
static int iRun = 0;
|
|
++ iRun;
|
|
svg_draw_polyline_chain("chain_polylines-initial", iRun, polylines);
|
|
#endif /* DEBUG_SVG_OUTPUT */
|
|
|
|
Polylines out;
|
|
if (! polylines.empty()) {
|
|
auto segment_end_point = [&polylines](size_t idx, bool first_point) -> const Point& { return first_point ? polylines[idx].first_point() : polylines[idx].last_point(); };
|
|
std::vector<std::pair<size_t, bool>> ordered = chain_segments_greedy2<Point, decltype(segment_end_point)>(segment_end_point, polylines.size(), start_near);
|
|
out.reserve(polylines.size());
|
|
for (auto &segment_and_reversal : ordered) {
|
|
out.emplace_back(std::move(polylines[segment_and_reversal.first]));
|
|
if (segment_and_reversal.second)
|
|
out.back().reverse();
|
|
}
|
|
if (out.size() > 1 && start_near == nullptr) {
|
|
improve_ordering_by_two_exchanges_with_segment_flipping(out, start_near != nullptr);
|
|
//improve_ordering_by_segment_flipping(out, start_near != nullptr);
|
|
}
|
|
}
|
|
|
|
#ifdef DEBUG_SVG_OUTPUT
|
|
svg_draw_polyline_chain("chain_polylines-final", iRun, out);
|
|
#endif /* DEBUG_SVG_OUTPUT */
|
|
return out;
|
|
}
|
|
|
|
template<class T> static inline T chain_path_items(const Points &points, const T &items)
|
|
{
|
|
auto segment_end_point = [&points](size_t idx, bool /* first_point */) -> const Point& { return points[idx]; };
|
|
std::vector<std::pair<size_t, bool>> ordered = chain_segments_greedy<Point, decltype(segment_end_point)>(segment_end_point, points.size(), nullptr);
|
|
T out;
|
|
out.reserve(items.size());
|
|
for (auto &segment_and_reversal : ordered)
|
|
out.emplace_back(items[segment_and_reversal.first]);
|
|
return out;
|
|
}
|
|
|
|
ClipperLib::PolyNodes chain_clipper_polynodes(const Points &points, const ClipperLib::PolyNodes &items)
|
|
{
|
|
return chain_path_items(points, items);
|
|
}
|
|
|
|
std::vector<const PrintInstance*> chain_print_object_instances(const Print &print)
|
|
{
|
|
// Order objects using a nearest neighbor search.
|
|
Points object_reference_points;
|
|
std::vector<std::pair<size_t, size_t>> instances;
|
|
for (size_t i = 0; i < print.objects().size(); ++ i) {
|
|
const PrintObject &object = *print.objects()[i];
|
|
for (size_t j = 0; j < object.instances().size(); ++ j) {
|
|
// Sliced PrintObjects are centered, object.instances()[j].shift is the center of the PrintObject in G-code coordinates.
|
|
object_reference_points.emplace_back(object.instances()[j].shift);
|
|
instances.emplace_back(i, j);
|
|
}
|
|
}
|
|
auto segment_end_point = [&object_reference_points](size_t idx, bool /* first_point */) -> const Point& { return object_reference_points[idx]; };
|
|
std::vector<std::pair<size_t, bool>> ordered = chain_segments_greedy<Point, decltype(segment_end_point)>(segment_end_point, instances.size(), nullptr);
|
|
std::vector<const PrintInstance*> out;
|
|
out.reserve(instances.size());
|
|
for (auto &segment_and_reversal : ordered) {
|
|
const std::pair<size_t, size_t> &inst = instances[segment_and_reversal.first];
|
|
out.emplace_back(&print.objects()[inst.first]->instances()[inst.second]);
|
|
}
|
|
return out;
|
|
}
|
|
|
|
Polylines chain_lines(const std::vector<Line> &lines, const double point_distance_epsilon)
|
|
{
|
|
// Create line end point lookup.
|
|
struct LineEnd {
|
|
LineEnd(const Line *line, bool start) : line(line), start(start) {}
|
|
const Line *line;
|
|
// Is it the start or end point?
|
|
bool start;
|
|
const Point& point() const { return start ? line->a : line->b; }
|
|
const Point& other_point() const { return start ? line->b : line->a; }
|
|
LineEnd other_end() const { return LineEnd(line, ! start); }
|
|
bool operator==(const LineEnd &rhs) const { return this->line == rhs.line && this->start == rhs.start; }
|
|
};
|
|
struct LineEndAccessor {
|
|
const Point* operator()(const LineEnd &pt) const { return &pt.point(); }
|
|
};
|
|
typedef ClosestPointInRadiusLookup<LineEnd, LineEndAccessor> ClosestPointLookupType;
|
|
ClosestPointLookupType closest_end_point_lookup(point_distance_epsilon);
|
|
for (const Line &line : lines) {
|
|
closest_end_point_lookup.insert(LineEnd(&line, true));
|
|
closest_end_point_lookup.insert(LineEnd(&line, false));
|
|
}
|
|
|
|
// Chain the lines.
|
|
std::vector<char> line_consumed(lines.size(), false);
|
|
static const double point_distance_epsilon2 = point_distance_epsilon * point_distance_epsilon;
|
|
Polylines out;
|
|
for (const Line &seed : lines)
|
|
if (! line_consumed[&seed - lines.data()]) {
|
|
line_consumed[&seed - lines.data()] = true;
|
|
closest_end_point_lookup.erase(LineEnd(&seed, false));
|
|
closest_end_point_lookup.erase(LineEnd(&seed, true));
|
|
Polyline pl { seed.a, seed.b };
|
|
for (size_t round = 0; round < 2; ++ round) {
|
|
for (;;) {
|
|
auto [line_end, dist2] = closest_end_point_lookup.find(pl.last_point());
|
|
if (line_end == nullptr || dist2 >= point_distance_epsilon2)
|
|
// Cannot extent in this direction.
|
|
break;
|
|
// Average the last point.
|
|
pl.points.back() = (0.5 * (pl.points.back().cast<double>() + line_end->point().cast<double>())).cast<coord_t>();
|
|
// and extend with the new line segment.
|
|
pl.points.emplace_back(line_end->other_point());
|
|
closest_end_point_lookup.erase(*line_end);
|
|
closest_end_point_lookup.erase(line_end->other_end());
|
|
line_consumed[line_end->line - lines.data()] = true;
|
|
}
|
|
// reverse and try the oter direction.
|
|
pl.reverse();
|
|
}
|
|
out.emplace_back(std::move(pl));
|
|
}
|
|
return out;
|
|
}
|
|
|
|
} // namespace Slic3r
|